Earth's surface water dives deep, transforming core's outer layer

Illustration of silica crystals coming out from the liquid metal of the Earth's outer core due to a water-induced chemical reaction. Image courtesy Dan Shim/ASU
Editor’s note: This story is featured in the 2023 year in review.
A few decades ago, seismologists imaging the deep planet identified a thin layer, just over a few hundred kilometers thick. The origin of this layer, known as the E prime layer, has been a mystery — until now.
An international team of researchers, including Arizona State University scientists Dan Shim, Taehyun Kim and Joseph O’Rourke of the School of Earth and Space Exploration, has revealed that water from the Earth's surface can penetrate deep into the planet, altering the composition of the outermost region of the metallic liquid core and creating a distinct, thin layer.
Their research was recently published in Nature Geoscience.
Research indicates that over billions of years, surface water has been transported deep into the Earth by descending, or subducted, tectonic plates. Upon reaching the core-mantle boundary, about 1,800 miles below the surface, this water triggers a profound chemical interaction, altering the core’s structure.
Along with Yong Jae Lee of Yonsei University in South Korea, Shim and his team have demonstrated through high-pressure experiments that subducted water chemically reacts with core materials. This reaction forms a hydrogen-rich, silicon-depleted layer, altering the topmost outer core region into a film-like structure. Additionally, the reaction generates silica crystals that rise and integrate into the mantle. This modified liquid metallic layer is predicted to be less dense, with reduced seismic velocities, in alignment with anomalous characteristics mapped by seismologists.
Illustration of Earth’s interior revealing subducting water and a rising plume of magma. At the interface where subducting water meets the core, a chemical exchange occurs to form a hydrogen-rich layer in the topmost outer core and dense silica in the bottom of the mantle. Image courtesy Yonsei University
“For years, it has been believed that material exchange between Earth's core and mantle is small. Yet, our recent high-pressure experiments reveal a different story. We found that when water reaches the core-mantle boundary, it reacts with silicon in the core, forming silica," said Shim. "This discovery, along with our previous observation of diamonds forming from water reacting with carbon in iron liquid under extreme pressure, points to a far more dynamic core-mantle interaction, suggesting substantial material exchange.”
This finding advances our understanding of Earth's internal processes, suggesting a more extensive global water cycle than previously recognized. The altered "film" of the core has profound implications for the geochemical cycles that connect the surface-water cycle with the deep metallic core.
This study was conducted by an international team of geoscientists using advanced experimental techniques at the Advanced Photon Source of Argonne National Lab and PETRA III of Deutsches Elektronen-Synchrotron in Germany to replicate the extreme conditions at the core-mantle boundary.
Members of the team and their key roles from ASU are Kim, who began this project as a visiting PhD student and is now a postdoctoral researcher at the School of Earth and Space Exploration; Shim, a professor at the School of Earth and Space Exploration, who spearheaded the high-pressure experimental work; and O’Rourke, an assistant professor at the School of Earth and Space Exploration, who performed computational simulations to comprehend the formation and persistence of the core's altered thin layer. Lee led the research team from Yonsei University, along with key research scientists Vitali Prakapenka and Stella Chariton at the Advanced Photon Source and Rachel Husband, Nico Giordano and Hanns-Peter Liermann at the Deutsches Elektronen-Synchrotron.
This work was supported by the NSF Earth Science program.
More Science and technology

Cracking the code of online computer science clubs
Experts believe that involvement in college clubs and organizations increases student retention and helps learners build valuable…
Consortium for Science, Policy & Outcomes celebrates 25 years
For Arizona State University's Consortium for Science, Policy & Outcomes (CSPO), recognizing the past is just as important as…
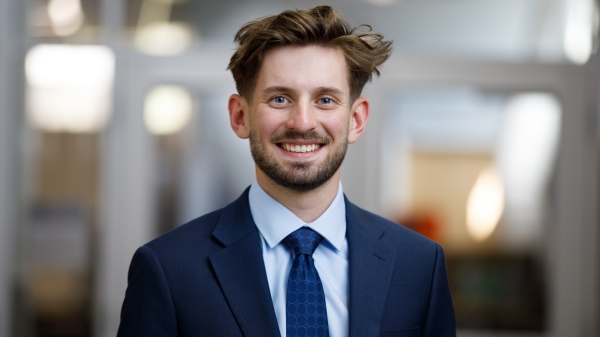
Hacking satellites to fix our oceans and shoot for the stars
By Preesha KumarFrom memory foam mattresses to the camera and GPS navigation on our phones, technology that was developed for…