Iron in ancient deep magma ocean probed by ultra-fast femtosecond X-ray lasers
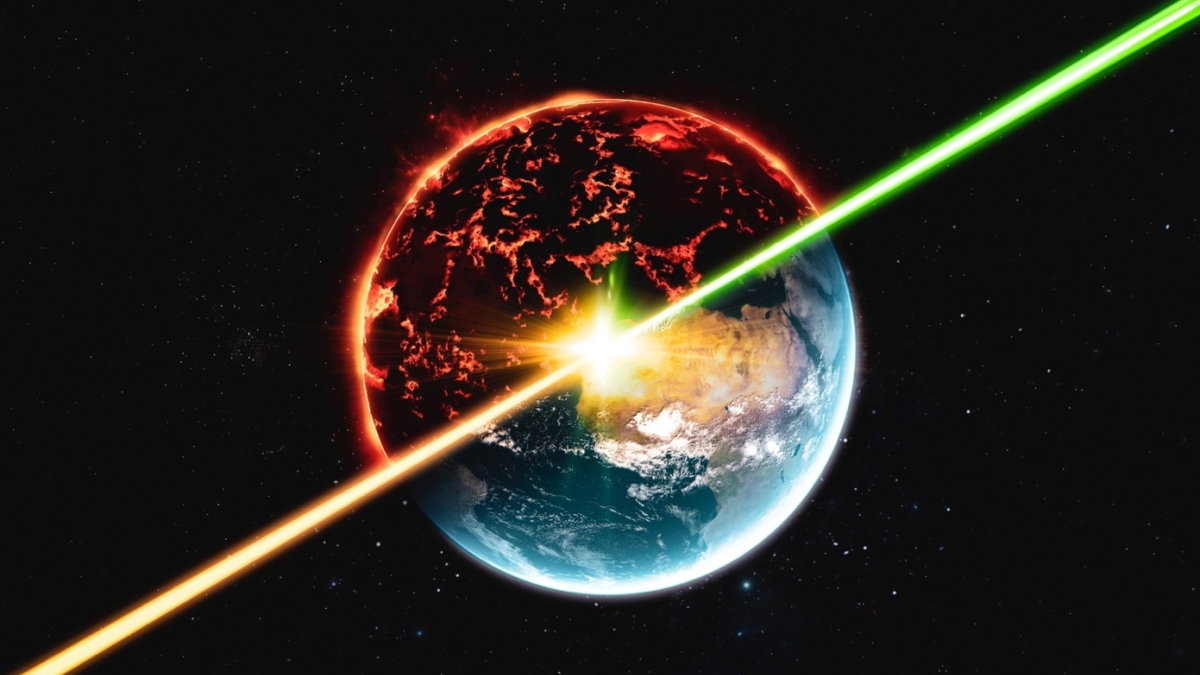
Image courtesy Greg Stewart/SLAC National Accelerator Laboratory
When the Earth was completing its formation approximately 4.5 billion years ago, it was enveloped in a global ocean of molten magma, extending hundreds to thousands of kilometers beneath its surface due to violent impacts. This early environment bore little resemblance to the habitable conditions we experience on Earth today. Nonetheless, these initial few hundred million years were crucial in shaping our planet's fundamental chemical and physical structures as we know them today. Key developments during this period included the formation of Earth's metal-rich core and silicate-rich mantle.
In the present-day Earth's mantle, recent seismic imaging studies have unveiled large mountain- to continent-sized enigmatic structures in the lowermost mantle. While the origin of these structures has been debated for over a decade, numerous models propose that these dense structures at the bottom of the mantle may be the last vestiges of the ancient global magma ocean.
A critical experimental observation supporting these models is that iron tends to concentrate more in the magma than the crystals at high pressures relevant to the lowermost mantle. This phenomenon renders the magma denser than the crystallized rocky layer, the mantle, during the late stages of mantle solidification, causing it to sink. Yet, a fundamental question persists: Why does iron preferentially dissolve into the magma rather than the crystals?
Many have postulated that the electronic structure of iron atoms may hold the key to this question. However, probing the electronic structure of iron atoms under such extreme conditions presents formidable challenges.
In pursuit of answers, an international team of scientists including ASU team members conducted experiments by subjecting silicate magma to pressures exceeding 1 million bars and temperatures of 7,000 degrees Fahrenheit using high-power laser beams. The results of their findings were recently published in Science Advances, with ASU School of Earth and Space Exploration geoscientist and Professor Dan Shim as lead author.
In this shock wave experiment, the sample can maintain a molten, highly compressed state for mere femtoseconds (a femtosecond is an extremely short amount of time, equivalent to one quadrillionth of a second). To investigate the electronic structure of iron atoms in silicate magma, which can only persist for such a brief time, they measured spectra from the iron atoms in the sample using ultra-fast, intense X-ray laser pulses at the Matter in Extreme Conditions (MEC) beamlines of Linac Coherent Light Source (LCLS), part of Stanford's SLAC National Accelerator Laboratory.
The experiments revealed that electrons in iron atoms rearrange into a configuration known as a low spin state, resulting in a denser state. This denser state is more stable for iron atoms under extreme pressures, making the magma a more favorable environment for iron atoms to be in. This atomic-scale transformation from ultra-fast experiments provides an explanation for why the magma can become denser than crystals as the magma ocean was solidifying, elucidating how the structures observed in seismic studies formed and persist in the deepest part of the mantle.
"In our experiments, we studied an event that happened 4.5 billion years ago, a super long time ago, 10^1710^17 seconds is a number with 17 zeros after it seconds ago. But to understand the ancient event, we used a laser-driven shock technique, which can make very high pressure for only a super tiny fraction of a second," Shim said. "The difference between the two time scales is mind-bogglingly huge (32 orders of magnitude)!"
Remarkably, these experiments also reached the pressure conditions expected to be found in super-Earth exoplanets, which are rocky planets beyond our solar system with radii one to 1.8 times greater than Earth's. The results suggest that magma densification could occur to a much larger extent in the magma oceans of super-Earths. Other studies have indicated that dense, iron-rich silicate magmas can generate dynamos at high pressures. Therefore, this study may contribute to understanding if silicate magmas in super-Earths may contribute to the generation of magnetic fields, potentially shielding the thin atmospheres of these planets from the intense radiation emitted by their host stars.
Additional authors on this study include Wendy Mao, Ariana Gleason and Roberto Alonso-Mori at Stanford University, Guillaume Morard at the University of Grenoble Alpes, and Alessandra Ravasio at the Laboratoire d'Utilisation des Lasers Intenses (LULI).
This work was supported by the NSF CSEDI Program and NASA Exoplanet Program.
More Science and technology
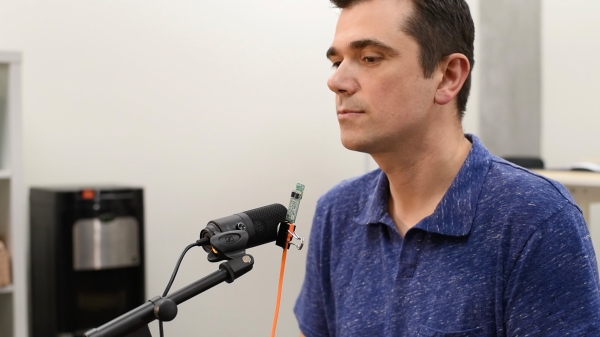
ASU researchers develop special microphone to verify human speech
Deepfakes have become a large societal concern with the advent of video and audio content generated by artificial intelligence,…
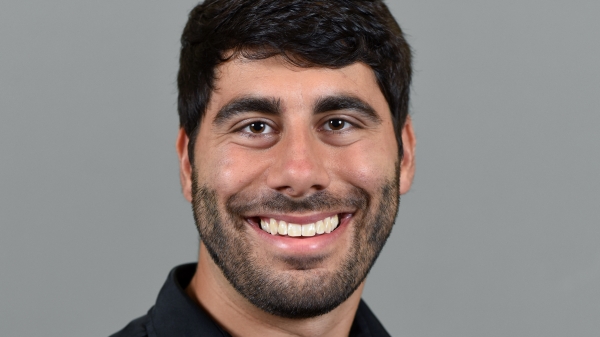
Leading students toward a future of renewable energy
Nicholas Rolston, assistant professor in the School of Electrical, Computer and Energy Engineering, one of the Ira A. Fulton…
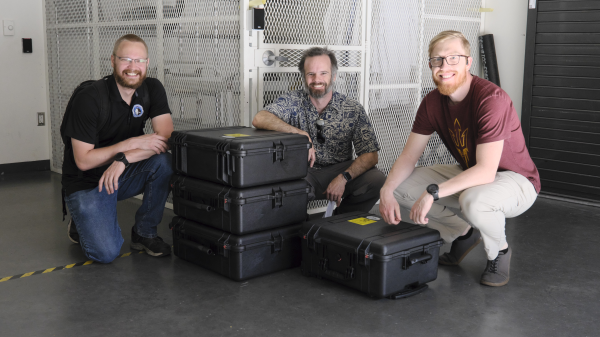
SPARCS mission spacecraft bus delivered to ASU for final assembly
The Arizona State University team that is building the NASA-funded Star-Planet Activity Research CubeSat, or SPARCS, cleared a…