A closer look at a deadly bacterium sets the stage for new vaccines
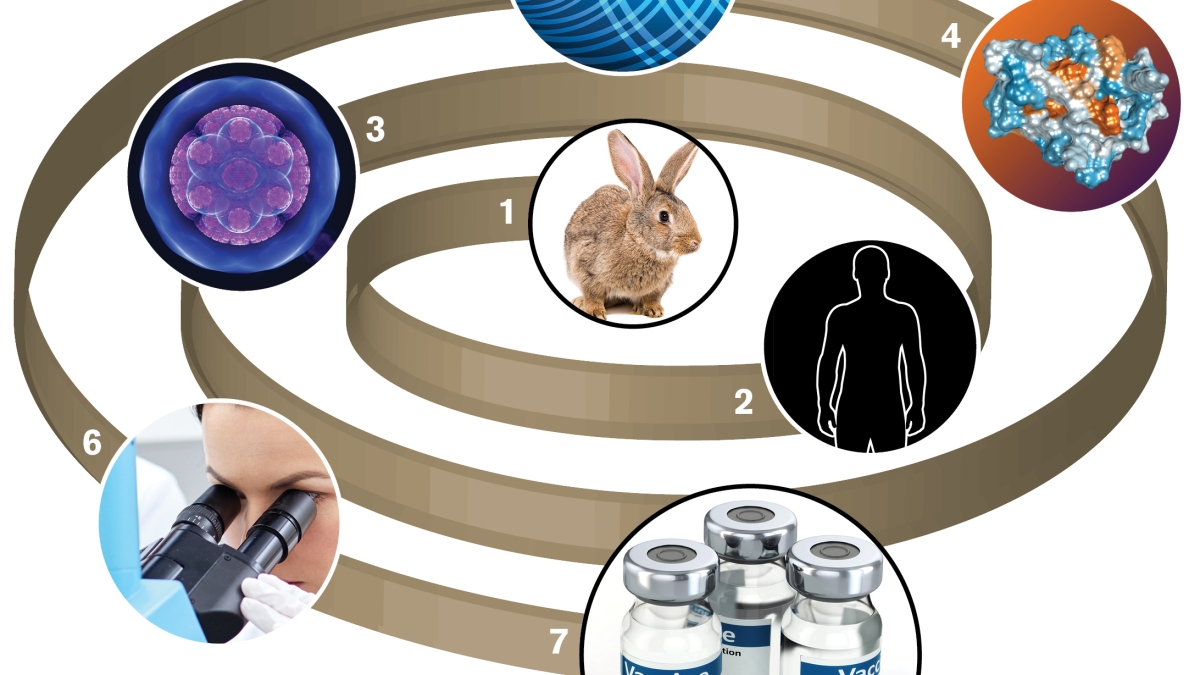
Tularemia, or rabbit fever, is a severely debilitating and sometimes fatal disease, and the pathogen involved has potential as a biological weapon. To better understand the disease – and to better design potential vaccines – an ASU team looked at a key cellular protein in unprecedented detail.
The bacterium Francisella tularensis is responsible for tularemia, which can spread from rabbits to people. In addition to being endemic in North America, Europe and Asia, the highly infectious pathogen is easily aerosolized, leading to its designation as a Class A bioterrorism agent.
In new research, Petra Fromme of Arizona State University’s Biodesign Institute and her ASU colleagues examine a key component responsible for the tularemia’s profoundly infectious character, using nuclear magnetic resonance spectroscopy (NMR) to explore features of the bacteria’s surface protein in unprecedented detail.
The study marks the first structural determination at atomic scale for any lipoprotein of the genus Francisella, and it holds the promise of improved therapies and potential vaccines.
According to James Zook of ASU’s Department of Chemistry and Biochemistry and lead author of the new study:
“Observing this virulence determinant at an atomic scale has shown us that this protein is a possible drug target. We now have an opportunity to develope a new, specialized pharmaceutical that specifically affects Francisella infections. This pathogen is a potential bioweapon; we want as many countermeasures as we can get.”
The group’s research findings appear in today’s issue of the journal Structure.
A natural (and man-made) threat
Tularemia is a spectrum of illnesses caused by F. tularensis, a bacterium existing in different subtypes bearing varying degrees of infectiousness. Rabbits, hares and pikas carry the most common strain of the bacteria, known as type A. The resulting disease is often referred to as rabbit fever.
The number of cases of tularemia in endemic regions is generally fairly low, with prevalence linked to areas of poor sanitation, lacking modern health care. Conflict zones are also vulnerable areas, with one of the largest modern outbreaks occurring in postwar Kosovo between October 1999 and May 2000, with 327 confirmed cases.
Vectors for the disease-causing bacterium include ticks, deer flies and other arthropods. After initial infection, tularemia undergoes an incubation period of one to 14 days, with most infections appearing after three to five days.
Symptoms of tularemia include loss of appetite, fever, lethargy and signs of sepsis – in severe cases leading to death. Additional symptoms include reddening and inflammation of the eyes and lymph node inflammation, which may be accompanied by suppuration (pus) and high fever, mimicking symptoms of plague.
The bacterium can enter the body through damaged skin and mucous membranes or through bacterial inhalation. Because F. tularensis in an intracellular pathogen, it can persist as a parasite within the cells of its host.
Typically, the bacterium infects white blood cells known as macrophages, using these as a refuge to evade recognition by the immune system. During the course of disease, the pathogen can spread to multiple organ systems, including the lungs, liver, spleen and lymphatic system.
F. tularensis has also been identified as a potential biological warfare agent by the Centers for Disease Control and Prevention. It has existed as a component in biowarfare projects in the United States, Russia and Japan at various times.
Several factors contribute to the bacterium’s attractiveness as a biological weapon, including the ease with which it can be aerosolized, its extremely infectious nature and the high degree of incapacitation produced in infected persons.
Vaccines needed
Treatment for tularemia is intensive and often unavailable in endemic regions, making vaccines the most attractive means of addressing the disease. Presently, there is a live attenuated vaccine available, though several factors limit its applicability. If improperly administered, the vaccine can induce infection when insufficient immunity is acquired following injection. This insufficiency leaves some 30-40 percent of a vaccinated population subject to infection.
For these reasons, the live vaccine strain, or LVS, vaccine for tularemia is not approved for use in the U.S. The authors of the current study therefore stress that an improved vaccine alternative is vitally needed.
The F. tularensis bacterium has a profound capacity for infection. Fewer than 10 cells are required and in some cases, a single bacillus is sufficient to cause disease. What accounts for this high propensity to cause disease or virulence? One answer, and the focus of the current study, is known as Flpp3, an outer membrane protein linked with aggressive infectiousness and implicated as a possible target of future vaccine efforts against tularemia.
Flpp3 is structurally related to a family of proteins known as Bet v1, which are responsible for many allergic reactions, including peanut and birch allergies. The fact that allergies caused by this protein family induce strong immune responses, suggests the related Flpp3 protein may be a promising target for vaccine and drug delivery efforts.
In earlier studies, a significant decrease in tularemia virulence was observed when a mutation was introduced into the DNA region coding for Flpp3, rendering the protein inoperative.
Probing with resonance
In the current study, nuclear magnetic resonance spectroscopy (NMR) was used to more closely home in on the membrane Flpp3. The protein examined with NMR was derived from a particularly virulent strain of tularensis, known as Type A SCHU S4.
The study marks the first atomic-scale structural investigation of this critical membrane protein. The precise function of Flpp3 remains obscure, and the authors suggest detailed structural analysis is the first step to unlocking this mystery.
Nuclear magnetic resonance for protein research (sometimes called protein NMR) is a powerful technique for revealing protein structure. It is similar in basic outline to magnetic resonance imaging (MRI) commonly used to examine human tissue in hospital settings, though protein NMR provides much higher sensitivity in order to capture structures more than a million times smaller than those studied by MRI radiologists. Here, at the scale of nanometers, molecular structure can be observed and characterized.
Protein NMR involves placing the sample inside a powerful magnet, passing radio-frequency signals through the sample, and measuring the level of absorption of these signals. Nuclei in individual atoms will absorb different radio frequencies, allowing researchers to determine distances between nuclei and compose detailed models of overall protein structure.
The study successfully determined the structure for Flpp3. The protein is largely spherical, containing a six-strand beta-sheet, two alpha-helices and three flexible loop regions. The NMR analysis revealed an internal binding pocket in the protein – a plausible target for vaccine and drug development.
In addition to uncovering structural details, the NMR study also provided new insights into the atomic-level dynamics of the protein, at rapid time scales. The authors suggest the loop structures of the protein may act like a gateway, blocking or enabling access to the protein’s internal binding cavity, depending on the variable conformation of these loops.
Ongoing research will further explore protein functionality, with particular focus on experimental and computational studies of drug activity.