Boosting seismic resilience

Liquefaction, which causes soils to act more like a liquid than a solid material, was the major cause of damage by the 2011 Christchurch earthquakes in New Zealand, which led to billions of dollars in damage and left more than 10,000 single family homes damaged beyond repair. ASU researchers have developed a new technique called microbially-induced desaturation to help limit damage from earthquake-induced liquefaction. Photo by Mark Lincoln/CC BY 2.0.
Still considered one of the most destructive natural disasters in U.S. history after more than a century, the San Francisco earthquake of 1906 lasted less than a minute. Despite the brief duration, its powerful shockwaves set off a chain reaction producing widespread and severe destruction for several days.
Several large fires ignited and blazed throughout the city. Some burned for three days. In all, the fires destroyed almost 500 city blocks. It’s been estimated that the fires and other impacts triggered by the intensity of the seismic jolt caused the death of approximately 3,000 people and left half of the city’s 400,000 residents homeless.
It wasn’t simply the sheer force of the ground shaking that led to catastrophic aftereffects, says Edward Kavazanjian, a Regents Professor of geotechnical engineering in the Ira A. Fulton Schools of Engineering at Arizona State University.
“The initial cause of much of the damage done by the San Francisco earthquake was due to extraordinarily extensive liquefaction that ruptured the city’s water lines,” Kavazanjian says. “So when the fires broke out around the city because of the quake, they didn’t have water to put the fires out.”
Liquefaction, as Kavazanjian explains it, happens when the ground turns into a viscous fluid. It can cause buildings to sink below the surface of the land they sit on, make buried water tanks pop up to the surface, and cause the ground to shift laterally and crack open.
“Liquefaction is the most damaging phenomenon that occurs in many earthquakes,” he says.
Kavazanjian notes that San Francisco’s public service facilities now include pumping systems that, in the event of an earthquake and the disruption of the city’s water supply systems, can pump water from the San Francisco Bay. Some neighborhoods also have large underground cisterns with reserve water supplies to be used in case of emergencies.
But more fully effective, sustainable and economically feasible solutions to protecting lives and property from earthquake-induced liquefaction are yet to be developed. And the need for such solutions is critical. That’s why Kavazanjian and fellow researchers have developed a new technique to lower the risk of soil liquefaction.
“Trillions of dollars’ worth of infrastructure around the world is at serious risk from even moderate earthquakes if substantial liquefaction occurs,” Kavazanjian says.
The 1964 earthquake in Niigata, a port city on Japan’s Honshu island, illustrates the extent of damage that can be done to buildings situated on soil saturated by liquefaction due to strong seismic shocks. Public domain image courtesy Joseph Penzien and the Japan National Committee on Earthquake Engineering
A ground treatment to hinder soil saturation
That risk is why the National Science Foundation is a major supporter of the kind of work being done by researchers like those at ASU’s Center for Bio-mediated and Bio-inspired Geotechnics, directed by Kavazanjian, and the Biodesign Swette Center for Environmental Biotechnology, directed by ASU Regents Professor of environmental engineering Bruce Rittmann. Kavazanjian and Rittmann are faculty members in the School of Sustainable Engineering and the Built Environment, part of the Fulton Schools, and members of the National Academy of Engineering.
Together, and at other times separately, Kavazanjian’s and Rittmann’s research teams have been involved in collaborations with other universities, industry partners, and local and regional government agencies to explore use of advances in engineering and science that might significantly reduce the harm that can be caused by liquefaction.
ASU Associate Research Professor Leon van Paassen is pictured installing a solar-powered wireless data logger that collects information provided by embedded sensors that measure hydraulic conductivity and water content of soil. The data is important for research aimed at developing methods of mitigating damage that can result from earthquakes. Photo courtesy the Center for Bio-mediated and Bio-inspired Geotechnics
Soil liquefaction occurs when a fully water-saturated soil is subject to strong shaking and liquefies, causing the soil to lose strength and resulting in especially hazardous ground failure.
Current efforts to hinder liquefaction focus predominantly on boosting what the experts call seismic resilience, which involves strengthening the ground and structurally reinforcing buildings, infrastructure and their surroundings to withstand the often violently forceful onslaught of shaking produced by earthquakes. Those protective processes can also help reduce damage from other kinds of environmental perils often triggered by strong seismic events.
One recent demonstration of the effectiveness of the processes involved work by Kavazanjian’s research group and Fulton Schools Associate Research Professor Leon van Paassen, along with Portland State University and University of Texas at Austin researchers, funded by the NSF’s Natural Hazards Engineering Research Infrastructure program.
The project explored an innovative ground treatment method developed by Center for Bio-mediated and Bio-inspired Geotechnics researchers to specifically reduce the potential for soil liquefaction without disturbing the ground. The treatment was applied at two testing sites in Oregon underlain by liquefiable soil, one in the vicinity of the Portland International Airport and one at the Port of Portland.
Work directed by van Paassen included the installation and interpretation of data derived by sensors that monitored the effects of the ground treatment process. He was aided by Fulton Schools graduate students Elizabeth Stallings Young and Caitlyn Hall. Two Center for Bio-mediated and Bio-inspired Geotechnics industry partners, Geosyntec Consultants and ConeTec, provided assistance in designing the treatment system and installation of the monitoring system.
Findings from the project led to further development of the ground treatment technique, with a focus on reducing the potential for liquefaction of soils under and around existing infrastructure.
The geotechnical engineering aspects of the project helped it earn a Western States Seismic Policy Council Award in Excellence for contributions to advances in preparing for, mitigating, responding to and recovering from earthquakes.
The 1989 earthquake in Loma Prieta, about 56 miles south of San Francisco, led to more than 60 deaths and injured almost 4,000 people, was felt in places hundreds of miles away from its epicenter and resulted in many scenes of shocking destruction. Photo by J.K. Nakata/U.S. Geological Survey
Microbes and bacteria can help lower risk of damage
The technique developed by the ASU team is called microbially-induced desaturation, or MID. It involves denitrification by naturally occurring microbes using a solution containing acetate and nitrate. The chemical reactions in the denitrification process yield nitrogen gas that displaces water in the soil’s pores.
Rittmann explains that when soils are injected with nitrate and acetate, naturally occurring bacteria in the soil consume those compounds and produce relatively large volumes of nitrogen gas in the soil, a process called biological denitrification. The gas forms tiny bubbles that lower the risk of soil liquefaction.
“This keeps the soil from losing its strength, so an earthquake will result in a lot less damage than would otherwise be done,” Rittmann says.
A recent news release from the American Geophysical Union provides more technical details on the process. Field trials and modeling of the method have shown it could help stabilize ground for at least two decades or longer before the treatment would need to be reapplied.
Researchers envision future implementation of the process involving the injection of the bubble-generating solution into soil beneath and around buildings and infrastructure systems, thereby stimulating the denitrifying microbes to fortify the ground against even some of the strongest seismic shocks.
The microbial aspects of this multifaceted biogeotechnical engineering venture, especially the denitrification process, are a focus of Rittmann’s environmental engineering work, particularly his center’s development of novel wastewater treatment methods.
Rittmann and his students, including Hall, developed mathematical biogeochemical models to understand in detail how MID generates the bubbles and to refine the recipe for adding nitrate and acetate to the soil to maximize effectiveness.
Kavazanjian and members of his team, chiefly then-graduate student Sean O’Donnell, took on the important task of modeling how the bubbles make the soil resistant to seismic force.
Stallings Young, Hall and O’Donnell applied the experience they gained on these projects to their work to later earn doctoral degrees through the Fulton Schools civil, environmental and sustainable engineering program.
In research supported by the National Science Foundation, Elizabeth Stallings Young (second from right) was among ASU graduate students who worked with Portland State University engineering faculty members and graduate students in recent years on extensive on-site studies of liquefaction. Photo by Leon van Paassen/ASU
A steep uphill path to full-scale operations
“Now the challenges are to validate our models, design the systems based on the models, take our technology out into the field and scale up MID for full-scale implementation,” Rittmann says.
It won’t be a one-size-fits-all solution, he adds, because not all soils have the same properties. The system will need to be adaptable to various ground conditions.
“We’ve shown in the laboratory that our mechanisms and processes can work, and we know how to implement them,” Rittmann says. “But we have to make it happen on a large scale, which is expensive research and will take a lot of work.”
But Kavazanjian and Rittmann say a look at the physical devastation, tragic loss of life and dire economic consequences resulting from events like the San Francisco earthquake — and even from less powerful quakes — should convince many that mounting technological defenses against such threatening seismic disruptions will ultimately be worth the labor and expense.
More Science and technology
Extreme HGTV: Students to learn how to design habitats for living, working in space
Architecture students at Arizona State University already learn how to design spaces for many kinds of environments, and now they…
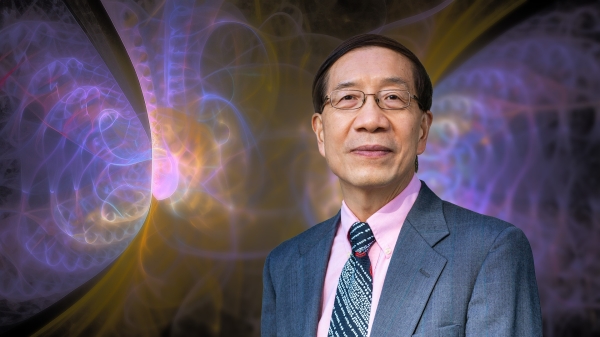
Human brains teach AI new skills
Artificial intelligence, or AI, is rapidly advancing, but it hasn’t yet outpaced human intelligence. Our brains’ capacity for…

Doctoral students cruise into roles as computer engineering innovators
Raha Moraffah is grateful for her experiences as a doctoral student in the School of Computing and Augmented Intelligence, part…