Sorry to burst your bubble, but helium is in short supply worldwide. This lighter-than-air gas is known for making party balloons float, but it has many other important uses, including medical imaging, making microchips and testing rockets.
To make the most of its supply, Arizona State University has equipped its Magnetic Resonance Research Center with a sustainable system that recycles the helium it uses for research. There, scientists are working to understand subjects like cancer, pain perception and degradable plastics.
The rise and fall of helium
For nearly a century, the U.S. has been the world’s biggest supplier of helium. While our helium industry was historically run by the federal government, it’s now transitioning into a private industry. Due to that transition and the shuttering of the Federal Helium Reserve, helium production has declined in the U.S. Now, this critical gas is increasingly coming from other countries like Qatar, Russia and Algeria, but the price and supply are not steady due to instability in these regions.
Helium is a non-renewable resource produced deep within the Earth as radioactive materials, like uranium, decay. The helium gradually bubbles its way up, and while most of it escapes the planet’s surface, a small portion becomes trapped under dense rock domes beneath the ground, where people can extract it.
Earth’s limited amount of helium is constantly shrinking, in part because helium gas is extremely hard to bottle up. Its structure is so small that we need special containers to hold it.
Think of birthday balloons, which we fill with a mix of helium and other gases. After a few days, the balloons are on the floor, even though they still appear to be full of air. That’s because all the helium has leaked out, leaving only the other gases behind.
Once helium escapes into the air, it passes through Earth’s atmosphere and goes into outer space, leaving the planet forever.
Despite its association with party decor and funny chipmunk voices, helium has a serious side. Its tiny size makes it perfect for testing leaks in rockets and gas pipelines. It’s not flammable, making it a safe choice for fabrication plants that have to build microchips in a sterile chamber filled with gas. And it can exist in liquid form near absolute zero (around minus 460 degrees Fahrenheit), the lowest possible temperature.
Its ability to stay super cold makes helium critical for magnetic resonance imaging machines. These MRI machines — known for taking detailed medical scans — rely on superconducting magnets. These special electromagnets must be kept extremely cold to work properly and are ruined if they rise above a certain temperature.
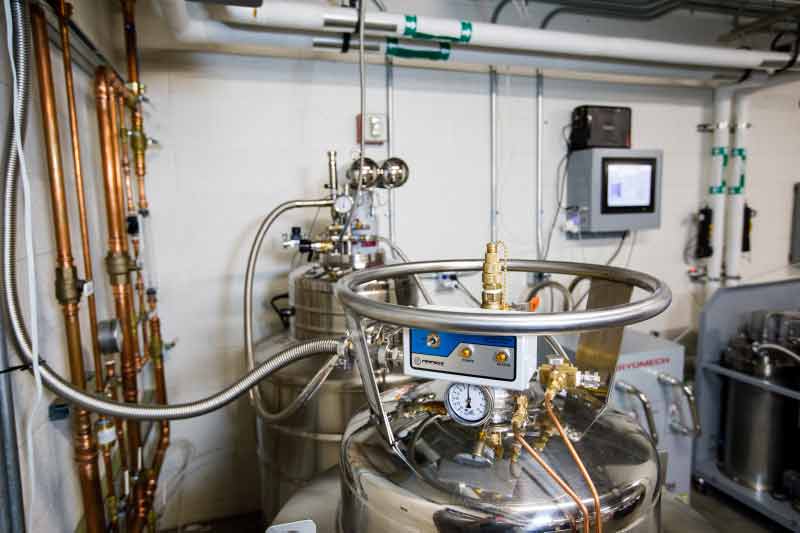
Machinery involved in recycling helium at the center, including a special transport tank in the foreground and a purifier, liquefier and compressor in the background. The center hopes to recover and recycle at least 85% of its helium. Photograph by Andy DeLisle/ASU
The long pipes to recovery
The ASU Magnetic Resonance Research Center relies on MRIs and nuclear magnetic resonance spectroscopy — like an MRI for atoms — to do its work, making helium a key resource.
To make the best use of its supply, the center, one of ASU’s Core Research Facilities, has installed a helium recovery system custom designed for its equipment and needs.
Inside the superconducting magnets, the liquid helium coolant gradually boils into a gas, which is captured and transported through networks of specially sealed copper pipes that give the lab a distillery look. The gas collects in giant bags that can hold up to 16,000 liters each. Once full, the gas in the bags is compressed and stored in mid-pressure tanks. From the tanks, the helium is sent to a purifier that removes any water vapor, oxygen or nitrogen before being converted to liquid, leaving the lab with pure liquid helium ready for reuse.
Each of ASU’s 16,000-liter helium collection bags holds enough gas to fill about 1,135 party balloons.
“Our long-term goal is to recover and recycle at least 85% of our helium,” says Brian Cherry, staff scientist and manager of the center. “Every five weeks, I have to fill one of my magnets, and if I don’t get helium within a day or two of that, $4 million of equipment is gone. So while we save money and recycle, we’re protecting that investment at the same time by not having to rely on a volatile market.”
Other universities have installed recovery systems of their own, but Cherry points out that ASU’s large storage tank system sets it somewhat apart from others. The center hopes to demonstrate the advantages of using a recovery system and inspire clinics, labs and other facilities to adopt similar systems and better preserve the world’s helium supply. One selling point: the tremendous cost savings.
"We’ve captured approximately 2,500 liters of liquid helium — nearly 2 million liters of helium gas — since June 2022," says Samrat Amin, the director of Core Research Facilities operations. At today's average price of liquid helium, the recovery system has already saved the center around $55,000.
"The cost savings with our helium recovery system represent a significant portion of our operation budget," adds Amin. "The system not only aligns with ASU’s sustainability goals, but also ensures our long-term ability to provide critical instruments that serve researchers across ASU."
The ASU recovery system will work in the background to support around 150 researchers — in addition to teaching labs, external businesses and collaborators — who use the core facility.
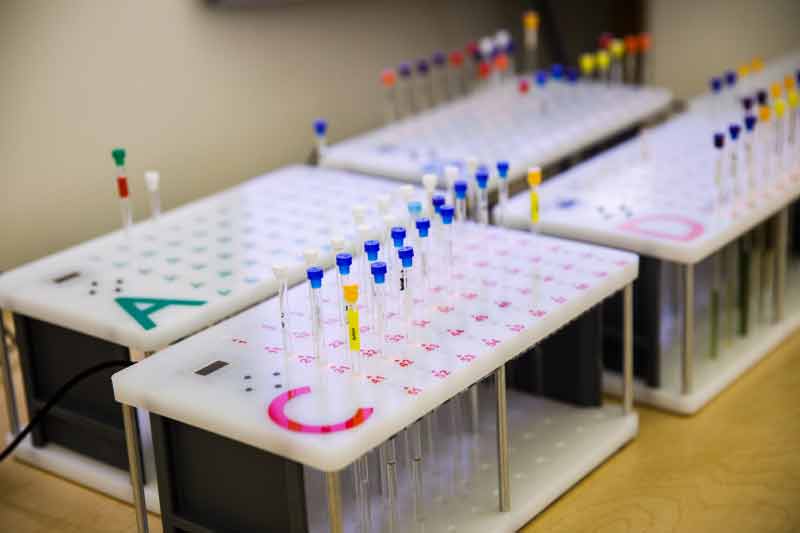
A rack of samples for NMR analysis. NMR imaging allows researchers to study molecules in solution, giving them a more realistic picture of how cells and proteins behave. Photograph by Andy DeLisle/ASU
Proteins shake
“I do everything using NMR spectroscopy, so the helium recovery system was absolutely crucial for me,” says Xu Wang, an associate professor in the School of Molecular Sciences who studies sugar-binding proteins.
These proteins are involved in a lot of biological processes. Wang has researched how sugar-binding proteins affect the symptoms of Lyme disease as well as the activation of white blood cells.
Most recently, his research group has been studying a sugar-binding protein produced by over 80% of brain tumor cells that influences tumors to grow more rapidly.
Associate Professor Wade Van Horn studies proteins found in the membranes that surround cells. These proteins carry information to and from the cell and are important for processes like drugs binding effectively to cells.
He is exploring how a new class of non-addictive pain medications interacts with cell receptors. The goal is to create ways to predict which drug variations will work best without relying on expensive clinical trials early on in the process.
There are other methods and machines for looking at molecular structures that don’t use superconducting magnets, and therefore helium. The trouble is that they require the molecules to stay in a fixed position and environment.
“What NMR does that’s different is that our studies are in solution,” Van Horn says. “We can see how the proteins wiggle around as they would in a biological context.”
That freedom of movement means that scientists can get a more realistic picture of how cells and proteins behave.
Because NMR machines have such a crucial role to play, the researchers can’t get around a need for helium. That’s why, when the National Institutes of Health announced funding for helium recovery systems for NMR facilities, Wang and Van Horn took the initiative to apply. Now, they’re able to continue using helium, and use it well.
“I think, in the very near future, we may not be able to acquire enough helium, even if we’re willing to pay increasing prices,” Wang says. “This helium recovery system will be crucial to the sustainability of the entire facility.”
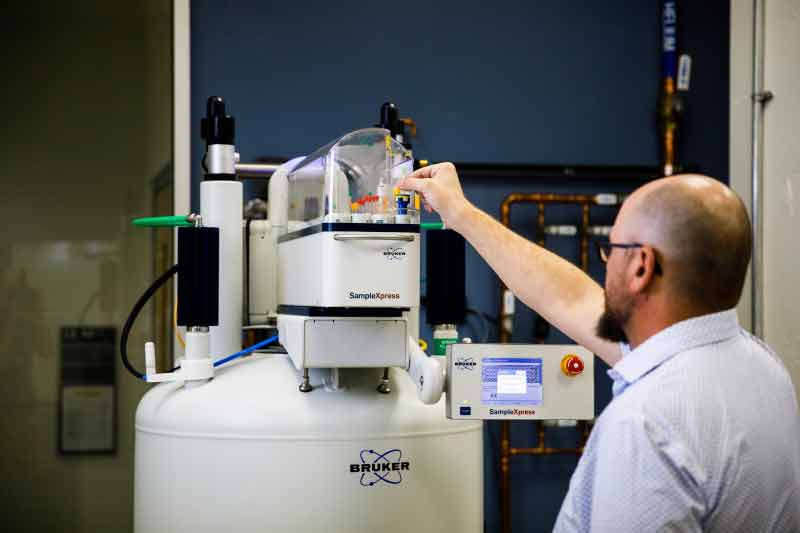
Brian Cherry, staff scientist and manager of the ASU Magnetic Resonance Research Center, loads samples into an automated NMR machine for analysis. Among other applications, scientists use NMR imaging for biomedical and materials research. Photograph by Andy DeLisle/ASU
Sustainable plastics, sustainably
It’s not only biomedical research that the recovery system supports. Timothy Long, a professor in the School of Molecular Sciences, uses the same machines as Van Horn and Wang to develop sustainable plastics and other materials.
According to the United Nations Environment Programme, people produce around 400 million tons of plastic waste each year. To address this environmental burden, the world sorely needs new kinds of green materials.
Long is working on creating polymers and composites that have desirable, sustainable qualities. The goal is to make plastics that come from nature-inspired molecules, need less source material and can be recycled easily while still performing as well as or better than traditional plastics.
“We use the Magnetic Resonance Research Center core facility every day, probably 600 times a month, and that facility is absolutely critical to what we're doing. NMR spectroscopy basically tells us the molecular structure of what we've made,” says Long, who is also the director of the Biodesign Center for Sustainable Macromolecular Materials and Manufacturing. “It's really the first experiment we need to do before we do anything else.”
The molecular structure of a material determines its physical properties, like durability. These properties then affect the performance of the material, like its ability to withstand harsh conditions. By looking at the molecular structure, Long can tell whether a material will be able to do what it needs to. Knowing the molecular structure also gives scientists a kind of proven recipe so that others can replicate the material.
“In my research center, we're worrying about the end of life of plastic, the sustainability of these materials. But we also need to be worried about the sustainability of the analytical tools that we use,” Long says. “I think this recycling system really exemplifies the university's focus on sustainability in every way.”
Helium in Arizona
Sustainability is about more than using resources wisely; it’s also about ensuring communities thrive in areas where we collect those resources.
Kirk Jalbert, an assistant professor in the School for the Future of Innovation in Society, studies public engagement with environmental science and policy. He recently worked with communities in northern Arizona to develop workshops that empower residents to make informed decisions about helium extraction in their area.
Geological surveys show that the Four Corners region would likely be a rich source of helium. Now that the helium extraction industry is shifting to privately owned operations, oil and gas companies have new incentives to use their equipment and expertise to turn from fossil fuels to helium. This means that there is potential for a new helium extraction industry in Arizona.
This could boost the state’s economy and benefit other critical industries like aeronautics and semiconductor manufacturing. However, extraction companies and communities must work together to ensure that those living near extraction sites benefit as well.
One way to encourage environmental justice, says Jalbert, is through community benefit agreements. These agreements make companies legally responsible for things like water protection, compensation for declining property value, subsidizing homeowners’ insurance, or making donations for schools or road improvements.
“The argument I make is that if this is required of these operators, then they know that they need to come into communities with a social obligation,” Jalbert says. “If we treated all of our rare resources that way, we would probably, as a society, be more likely to account for the real value of those resources.”
The helium recovery system and research efforts described in this story were partially funded by the National Institute of General Medical Sciences, part of the National Institutes of Health.
Top illustration by Hannah Kalas
More Science and technology

How ASU is reshaping manufacturing training
The manufacturing sector faces a persistent challenge: a shortage of skilled workers.As industries adopt emerging technologies, traditional training methods struggle to meet the demand for skilled…

Podcast explores the future in a rapidly evolving world
What will it mean to be human in the future? Who owns data and who owns us? Can machines think?These are some of the questions pondered on a newly launched podcast titled “Modem Futura.” Co-…
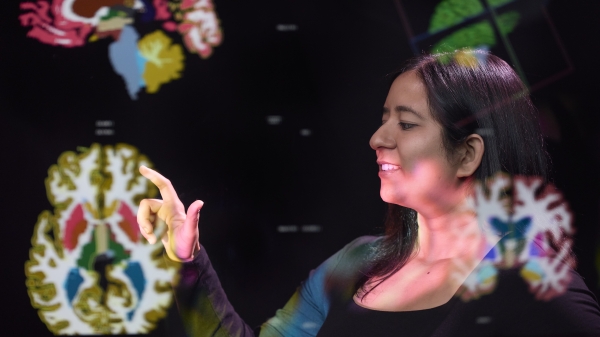
New NIH-funded program will train ASU students for the future of AI-powered medicine
The medical sector is increasingly exploring the use of artificial intelligence, or AI, to make health care more affordable and to improve patient outcomes, but new programs are needed to train…