Ray Carpenter retires after four-decade distinguished career at ASU
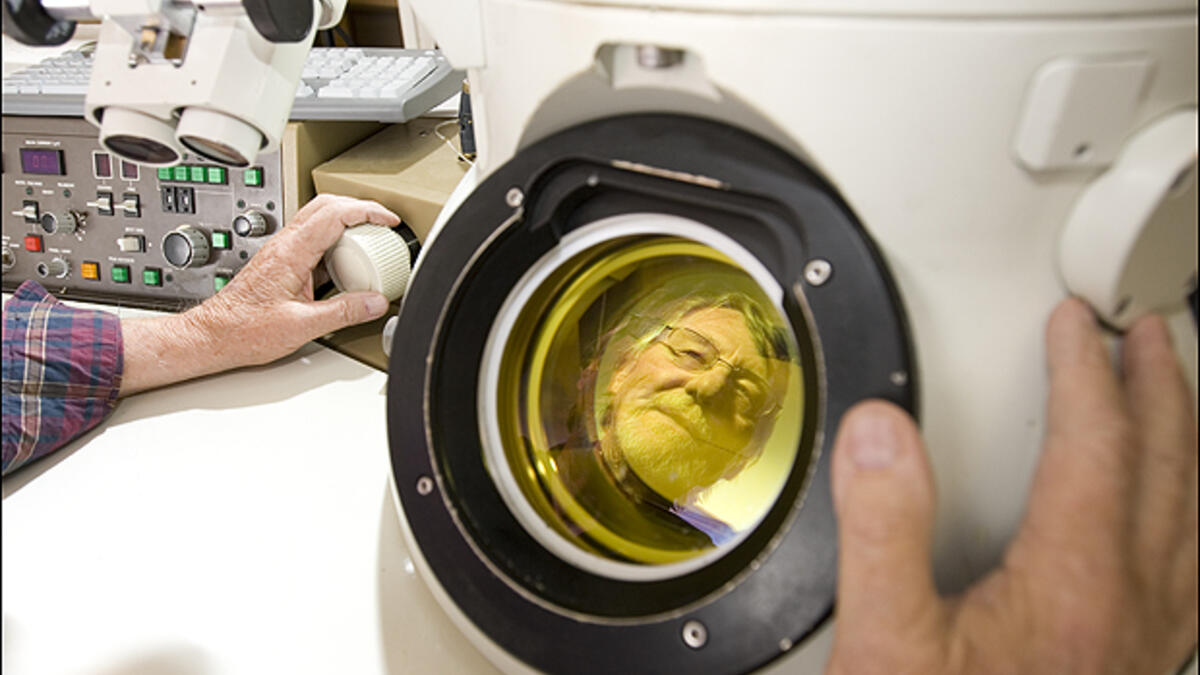
Ray Carpenter reflects on his ASU career.
The past four decades have seen many advances in electron microscopy at Arizona State University, and Ray Carpenter has been instrumental in many of them.
After a productive 13 years at Oak Ridge National Laboratory, Carpenter came to ASU in 1980 as a tenured full professor and the founding director of the NSF National Facility for High Resolution Electron Microscopy in the interdisciplinary Center for Solid State Science.
Last week, Carpenter gathered with colleagues, former students, friends and family at ASU’s University Club to celebrate his career.
“When I look back over my career, I have to say my whole time at ASU has been quite memorable," said the professor in the School of Molecular Sciences and the Eyring Materials Center. "Through the center and its Winter Schools program and research conferences, I have interacted and worked with internally known researchers who are quite prominent experts in the electron microscopy/solid state science world. Being able to interact with such a large group of accomplished scientists, engineers and students with such strong common interests is a memorable event spanning decades.”
Carpenter’s career has also been remarkable. He took the lead in helping ASU acquire two aberration-corrected electron microscopes, which were funded by a large Major Research Instrumentation grant from the National Science Foundation. Installation of these instruments was no small feat as it required the design and construction of a building without stray magnetic or electric fields, nor mechanical or acoustic vibrations that would distort images and degrade resolution.
“The measured stage drift rates of both microscopes were about 1,000 times less than continental drift rates, which is quite remarkable,” Carpenter said. “These microscopes made atomic resolution straightforward and continue to produce striking research results.”
In addition to research, Carpenter enjoyed teaching students practical applications of electron microscopy. Carpenter, with longtime colleague David Smith, developed and taught a comprehensive Transmission Electron Microscope (TEM) course with applications to physics, chemistry, geology and materials science.
“When I joined ASU, the dean of engineering requested that I teach a microscopy class that was useful to engineers,” Carpenter said. “The course, which included an emphasis on applications to materials problems, began as a one-semester lecture class. With the help of Professor Dave Smith, the course expanded to fall and spring lecture classes with three-credit laboratory classes with hands-on microscope for students, totaling 12 credits in microscopy.”
David Smith, who taught the second-semester TEM class, said, “Hundreds of students have taken Ray’s TEM class, and many of them now hold faculty positions not only around this country, but also around the world. These classes became the most comprehensive electron-microscopy courses in the country, if not the world. Many of our microscopy students have also gone on to distinguished careers in national labs and industry.”
Carpenter has been active at many levels throughout the university, including serving as director of the Center for Solid State Science, and chairing and serving on many PhD committees. Carpenter was also the founding director of the Science and Engineering of Materials graduate program. His many accomplishments were recognized with the ASU Faculty Achievement Award in 1990.
Outside of ASU, Carpenter has been active in several professional societies throughout his career, primarily the Microscopy Society of America, where he is a member of the inaugural class of elected fellows. Carpenter also served as director of Physical Sciences of the Microscopy Society of America, and he was later elected as its president.
Retirement for Carpenter will include some continued research at ASU but will incorporate more time with family and traveling.
“I enjoy family gatherings during holiday, birthdays and other celebrations,” Carpenter said. “I look forward to enjoying our national parks and events like chuckwagon cookouts and dude ranches. There are lots of things to do.”
More Science and technology

Lucy's lasting legacy: Donald Johanson reflects on the discovery of a lifetime
Fifty years ago, in the dusty hills of Hadar, Ethiopia, a young paleoanthropologist, Donald Johanson, discovered what would…

ASU and Deca Technologies selected to lead $100M SHIELD USA project to strengthen U.S. semiconductor packaging capabilities
The National Institute of Standards and Technology — part of the U.S. Department of Commerce — announced today that it plans to…
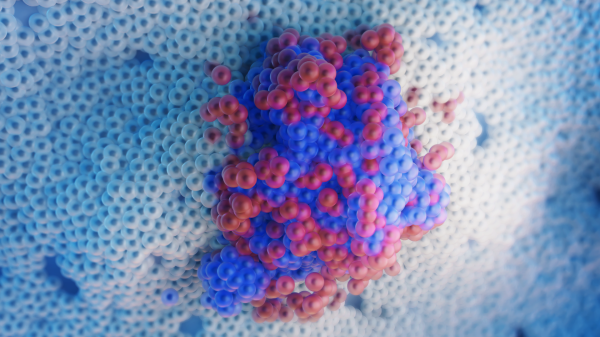
From food crops to cancer clinics: Lessons in extermination resistance
Just as crop-devouring insects evolve to resist pesticides, cancer cells can increase their lethality by developing resistance to…