Lost in the moss: ASU scientists answer key questions regarding photosynthesis evolution
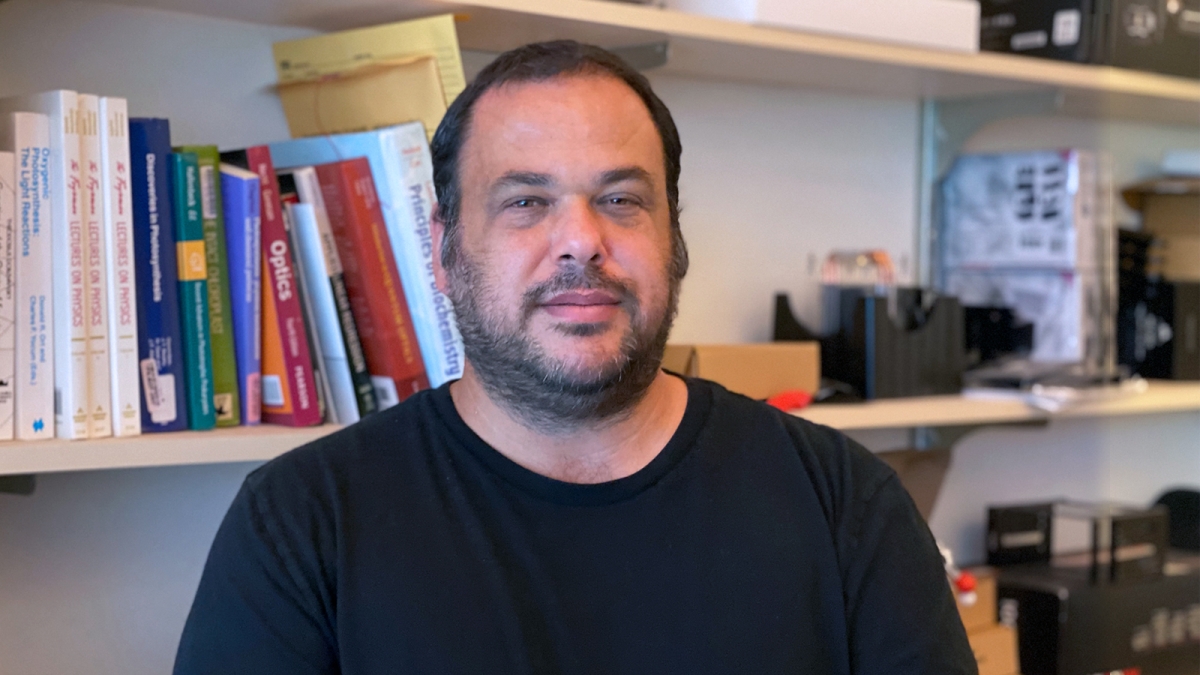
Yuval Mazor is an assistant professor in the School of Molecular Sciences and the Biodesign Institute at ASU. Photo by Mary Zhu/ASU
A team of scientists from Arizona State University has taken a significant step closer to unlocking the secrets of photosynthesis, by determining the structure of a very large photosynthetic supercomplex in mosses.
This important discovery is laid out in their paper just published in Nature Plants. The paper is titled "The structure of the Physcomitrium patens photosystem I reveals a unique Lhca2a paralogue replacing Lhca4."
“This structure is a major breakthrough in tracing the evolutionary path of photosystems and their antennae along the green lineage leading to modern-day plants,” said Yuval Mazor, the paper’s senior author and an assistant professor in the School of Molecular Sciences and the Biodesign Institute’s Center for Applied Structural Discovery.
Understanding photosynthesis
Oxygenic photosynthesis, responsible for the biosphere as we know it today, evolved in cyanobacteria 2.5 billion years ago.
The incorporation of cyanobacteria into a non-photosynthetic eukaryotic cell established the green lineage of photosynthetic organisms leading to higher plants close to 1.5 billion years ago. About 450 million years ago, the ancestors of present-day plants began colonizing dry land, making moss the best representative of this evolutionary process.
The transition from a marine to a terrestrial environment brought with it a large increase in light intensity and desiccation without the shielding effects of water. These environmental changes resulted in adaptations in the photosynthetic machinery of the first land colonizers. Most of the adaptations in the light reactions occurred as different antennae systems evolved to change light harvesting and photoprotection pathways.
"Mosses are a basal member of the plant family tree and represent a unique period of plant evolution,” said Chris Gorski, graduate student and one of three first authors on the paper. “Our work sheds light on the adaptations made to light harvesting and photosystem I composition by some of these early plant ancestors after the transition from a marine environment to a harsher terrestrial one."
From left to right: Alan Nisanov, undergraduate research student; Christopher Gorski, grad student; Zhen Da, grad student; Assistant Professor Yuval Mazor; Zach Dobson, grad student; Jin Li, grad student; Halima Khatun, grad student; and Randel Maqdisi, undergraduate research student. Photo by Mary Zhu/ASU
As stated, mosses are important as a stepping stone to land adaptation as part of the evolution of vascular, flowering plants. Mosses can live on dry land as well as in aquatic environments. They don’t have the vascular tissues and embryos of higher plants but are multicellular and their life cycle is the basis for more “advanced” plants.
In this paper, Mazor and his team describe the cryogenic electron microscopy (cryo-EM) structure of the moss Photosystem I together with its LHCI antenna at 2.8 Å (an angstrom is a tenth of a nanometer, which in turn is a millionth of a millimeter) resolution and its peripheral antenna (called LHCI) in mosses.
A very distinct characteristic of vascular plants — all plants that evolved “after” mosses — is that they contain low energy chlorophylls in LHCI. These low energy chlorophylls, which are “redder” than regular chlorophylls, are carried on a gene called Lhca4 (part of LHCI).
This moss does not have Lhca4 genes but has a large number of similar genes. In the team’s structure, they were able to identify the specific gene replacing Lhca4. Because Lhca4 genes exist in organisms that predate mosses, they think this branch of mosses have lost their Lhca4 and replaced it with the newly identified gene (called lhca2b).
This is significant because it shows that the light absorption properties of LHCI (low energy chlorophylls absent or present) can change within a relatively short time. Mazor thinks this adaptation relates to the ability of mosses to maintain their ability to grow underwater where low energy light (red shifted) is scarce due to water column absorption.
In the past few years, single-particle cryo-EM in particular has triggered a revolution in structural biology and has become a newly dominant discipline. Cryo-EM allows researchers to take a look at biological structures that were simply not accessible just a few years ago, and is now exposing structures of unprecedented complexity in great detail.
Indeed, it is this technique utilized by the experts in the School of Molecular Sciences and The College of Liberal Arts and Sciences that has enabled the elucidation of the structure of the PSI in mosses.
Mazor’s team includes Gorski, research technician Reece Riddle and post-doctoral fellow Hila Toporik, all first co-authors. Graduate students Zhen Da and Zach Dobson, as well as Dewight Williams, an associate research scientist in the John M. Cowley Center for High-Resolution Electron Microscopy, also made major contributions to the study.
The authors would like to acknowledge the use of the Titan Krios at the Erying Materials Center at Arizona State University and the funding of this instrument by NSF MRI 1531991. This study is funded by a startup grant from Arizona State University and supported by grant number 2034021 from the National Science Foundation to Mazor.
More Science and technology
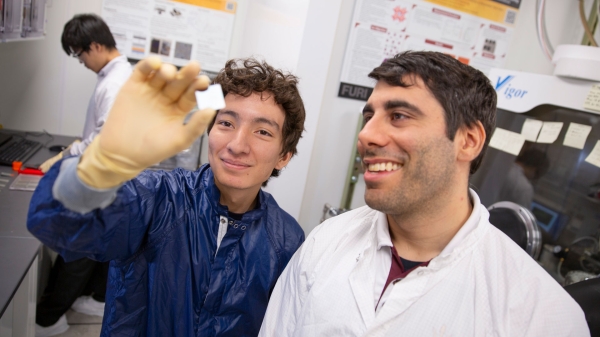
ASU student researchers get early, hands-on experience in engineering research
Using computer science to aid endangered species reintroduction, enhance software engineering education and improve semiconductor…

ASU professor honored with prestigious award for being a cybersecurity trailblazer
At first, he thought it was a drill.On Sept. 11, 2001, Gail-Joon Ahn sat in a conference room in Fort Meade, Maryland.…

Training stellar students to secure semiconductors
In the wetlands of King’s Bay, Georgia, the sail of a nuclear-powered Trident II Submarine laden with sophisticated computer…