Improvements in microscopy home in on biology's elusive details

The ASU research group (from left): Abhishek Singharoy, John Vant, Jonathan Nguyen, Petra Fromme, Chitrak Gupta and Wade Van Horn.
In the late 1600s, the Dutch tradesman Antonie van Leeuwenhoek began investigating the world of the very small using the first microscope, discovering a riotous world of protists, bacteria and other previously unseen organisms. Subsequent generations of scientists have developed ever more sophisticated means of probing the microscopic world, bringing many mysteries of the biological realm into stunning relief.
Now, researchers at Arizona State University's Biodesign Center for Applied Structural Discovery and School of Molecular Sciences, as part of a multi-institutional research collaboration, are carrying the field of microscopy a step further, refining a technique known as cryogenic electron microscopy, or cryo-EM.
The technology involves flash-freezing a biological sample of interest, then using a beam of electrons to image and record thousands of 2D images, assembled by means of computer into an atomic profile of the sample’s structure. Such density maps, as they are known, can then be converted into a detailed 3D image.
The method is particularly useful for ferreting out subtleties of protein structure, which are often missed through conventional modelling strategies. Such information is critical for the understanding of health and disease. Since proteins are the primary targets of most pharmaceutical drugs, a fuller picture of their structure and function is essential for designing more effective treatments, with fewer side effects.
The new research describes a method for producing more accurate structures, through a sophisticated statistical method known as maximum entropy. This approach — which has been effectively applied in many domains ranging from protein research and neuroscience to ecology and the behavior of animal populations — is ideally suited to the refinement of cryo-EM data, producing the most unbiased structural model of a biological sample.
Molecules like proteins assume complex, three-dimensional forms and can also change shape during their functioning.
“Complex biomolecules actually exist in an ensemble of states, and you can take a kind of snapshot of these molecules in different conformations,” said Abhishek Singharoy, corresponding author of the new study and an assistant professor at ASU.
Some of these conformations may persist in time, but others are extremely ephemeral, coming and going on time scales measured in billionths of a second.
The new technique described allows researchers to model these transitory structures, which can play a vital role in biological processes but are often missed using traditional cryo-EM techniques.
The ASU team is joined by researchers from the University of Illinois; Purdue University; Department of Mathematics and Computer Sciences, Grenoble, France; the University of Florida; and Stony Brook University.
“This work highlights how the integration and streamlining tools developed by labs with complementary expertise can be used in combination with experimental data to advance our understanding of structural biology,” said Alberto Perez of the University of Florida.
The group’s findings appear in the current Cell Press journal Matter, appearing on the journal's cover.
One of a triad of methods
A range of modern imaging techniques allows researchers to investigate crucial molecules of life, including proteins, nucleic acids and even single molecules. Cryo-EM, a variant of electron microscopy, was first developed in the 1970s. The technique was later recognized with the the 2017 Nobel Prize in chemistry, awarded to Jacques Dubochet, Joachim Frank and Richard Henderson, pioneers in the field, for developing cryo-electron microscopy for the high-resolution structure determination of biomolecules in solution.
Like other forms of electron microscopy, cryo-EM replaces photons of light used for illuminating samples in conventional light microscopy with a beam of electrons. Because resolution of an object by microscopy is limited to roughly one-half the wavelength of light used to illuminate the sample, the shorter wavelengths of electrons, which are dependent on their momentum, enable scientists to visualize tiny structures with astonishing clarity.
Cryo-EM is one of a triad of methods used for research in structural biology, joining X-ray crystallography and nuclear magnetic resonance spectroscopy (NMR). Each method has its own strengths. While X-ray crystallography can produce stunningly detailed structures in very high resolution, especially using X-ray free electron lasers or XFEL technology, the method struggles to image large or complex structures, including membrane proteins, as they are challenging to crystalize. The same is true of NMR.
Cryo-EM comes of age
Imaging large, complex biomolecules is where cryo-EM hits its stride, because samples do not require crystallization and can be studied in their native surrounding environment. In recent years, a new generation of high-speed cameras have been developed to help capture the dynamic activity of various biomolecules. The tricky part arises when researchers try to produce a 3D structural model from the raw data provided in the initial 2D density map of the molecule. Previously, this has involved making an educated guess about what the structure may look like and fitting the information provided in the density map into this model.
Inaccuracies can arise from the overfitting of raw data into the structural model. The new approach instead makes no assumptions about the final molecular structure apart from constraints that are known with certainty. By producing the most unbiased structure, this maximum entropy approach can help researchers fill in the blanks during structural determination, better accounting for the contribution of various conformations that may exist at very low frequency. A full understanding of biomolecules like proteins requires the simultaneous determination of the structures of all the relevant states that these molecules can assume.
To visualize this in simple terms, imagine trying to produce a model that illustrates the behavior of a boy who is standing still for an hour, except for occasional, fleeting movements of arms and legs. On average, nothing changes, and a resulting model of the boy would consist of a static, motionless image. A maximum entropy approach on the other hand would allow all various gestures, however brief, to contribute to the final image, yielding a much more accurate representation.
The new study presents six examples of elaborately folded proteins of various sizes, including large membrane and multi-domain systems. The results emphasize the ability of a maximum entropy statistical package known as CryoFold to discover molecular ensembles, including rare low-probability structures that have been experimentally validated and recognized as functionally relevant.
The maximum entropy technique can be used in conjunction with existing methods of data fitting in an iterative process capable of turning low-resolution data into high-resolution, 3D structures with a high degree of confidence. Such advances are helping cryo-EM reach its full potential by characterizing the entire conformational landscape of proteins and other important biomolecules.
“This work integrates multiple physics-based approaches to refine protein structures from cryo-EM data, providing not a single, static image of the protein, but rather a collection of structures, which is more representative of the true, dynamic nature of proteins,” said Chitrak Gupta, co-author and a researcher in the Center for Applied Structural Discovery and School of Molecular Sciences.
More Science and technology

Cracking the code of online computer science clubs
Experts believe that involvement in college clubs and organizations increases student retention and helps learners build valuable…
Consortium for Science, Policy & Outcomes celebrates 25 years
For Arizona State University's Consortium for Science, Policy & Outcomes (CSPO), recognizing the past is just as important as…
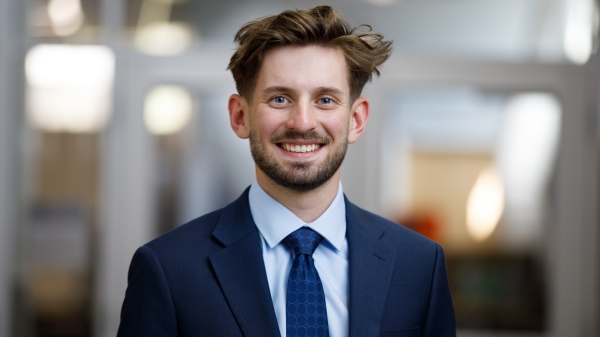
Hacking satellites to fix our oceans and shoot for the stars
By Preesha KumarFrom memory foam mattresses to the camera and GPS navigation on our phones, technology that was developed for…