Computational protein design utilizes unnatural amino acids

Assistant Professor Jeremy Mills from ASU’s School of Molecular Sciences and the Biodesign Institute’s Center for Molecular Design and Biomimetics.
Proteins are the molecular machines that make all living things hum. They stop deadly infections, heal cells, capture energy from the sun and so much more.
Proteins are built by stringing together chemical building blocks called amino acids, according to instructions in an organism’s genome. These strings then “fold up” based on the chemical forces between the amino acids, forming the complex three-dimensional structures needed to perform specific jobs.
Fluorescent proteins have revolutionized our ability to study biological systems. Despite a wealth of fluorescence tools, some fundamental biological processes — like the interactions between proteins and metabolites — remain difficult to study.
Assistant Professor Jeremy Mills and his group in Arizona State University's School of Molecular Sciences and the Center for Molecular Design and Biomimetics in the Biodesign Institute have just published their research in the journal Biochemistry and present a novel solution to this problem.
Specifically, they use a fluorescent amino acid that is not found in nature to generate a number of new fluorescent proteins whose light-emitting properties change when they interact with biotin, a compound that is very important in a number of metabolic processes.
“An important aspect of this study is that atomic-level pictures of many of these new proteins were generated that provide a great deal of information about how the binding of biotin changes the fluorescence properties of the proteins,” Mills said. “This information lays a foundation for the development of new fluorescent proteins that will help further the legacy that fluorescent proteins have already forged in the study of biological systems.”
“The protein studies of Jeremy Mills are typified by outstanding scholarship and a relentless commitment to making critical advances that will benefit science and society at large,” said Tijana Rajh, director of the School of Molecular Sciences.
This study involved a huge amount of work, including designing protein constructs and experiments, and purifying and crystalizing the proteins in order to collect the diffraction data. The data will be used in future studies aimed at the rational design of fluorescent, protein-based sensors of small molecule binding or dissociation.
This work was funded by a grant from the National Institutes of Health (NIH). The Research Project Grant (R01) is the original and historically oldest grant mechanism used by NIH. The focus of the grant is to develop novel fluorescent, protein-based tools that will be broadly applicable to the study of biological systems in ways that would be very difficult to achieve using existing fluorescent proteins.
Although nature has been building proteins for more than three billion years, the number of possible proteins is astronomical: There are more ways of assembling 100 amino acids than there are atoms in the universe. For years, scientists have tried to predict the shapes protein molecules should assume based on their amino acids — with limited success. The current study is a significant step toward understanding how to harness the power of proteins to help guide future efforts to rationally design new fluorescent sensors.
Mills is also passionate about bringing his enjoyment of science to the local community. He attributes a great deal of his love of research to participation in science fairs at the local and international levels while in high school. He serves as a judge at the Arizona Science and Engineering Fair and the International Science and Engineering Fair when possible. He also frequently demonstrates the use of the protein folding software Foldit to the public and has even developed lessons that are aimed at lay audiences that use Foldit as their foundation.
The team from the School of Molecular Sciences and the Biodesign Center for Molecular Design and Biomimetics also includes: Patrick Gleason, Bethany Kolbaba-Kartchner, Nathan Henderson, Erik Stahl and Chad Simmons.
More Science and technology
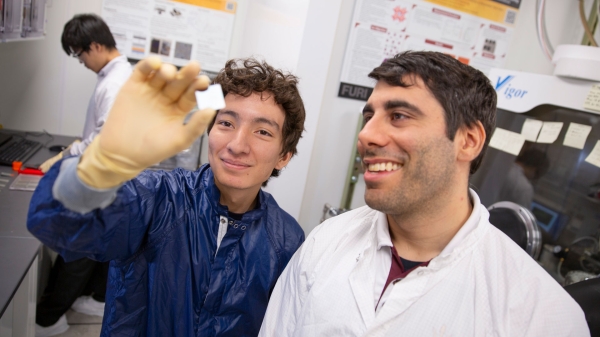
ASU student researchers get early, hands-on experience in engineering research
Using computer science to aid endangered species reintroduction, enhance software engineering education and improve semiconductor…

ASU professor honored with prestigious award for being a cybersecurity trailblazer
At first, he thought it was a drill.On Sept. 11, 2001, Gail-Joon Ahn sat in a conference room in Fort Meade, Maryland.…

Training stellar students to secure semiconductors
In the wetlands of King’s Bay, Georgia, the sail of a nuclear-powered Trident II Submarine laden with sophisticated computer…