ASU engineers get to the heart of organs-on-a-chip
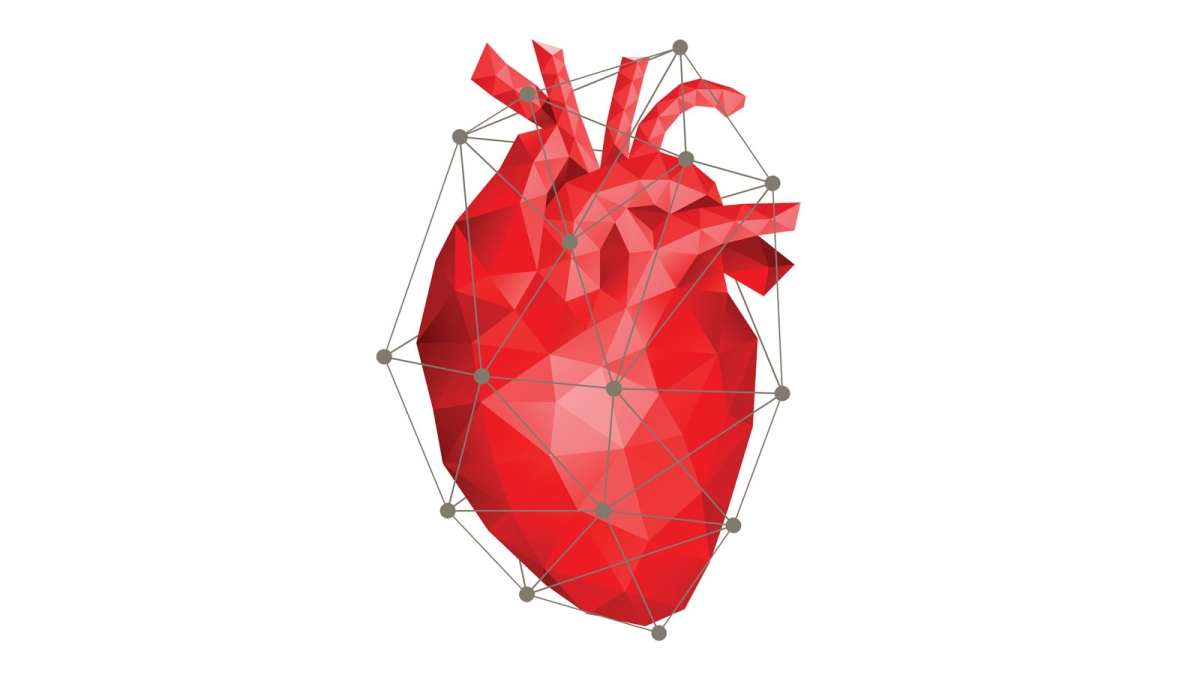
A polygonal graphic of the human heart. Arizona State University biomedical engineering doctoral student Jaimeson “Jaime” Veldhuizen and Associate Professor Mehdi Nikkhah collaborated with an interdisciplinary research team to design and validate a new heart-on-a-chip platform for use in disease modeling, drug testing and other research. Graphic courtesy of Shutterstock
Providing meticulous care of the human heart means having an accurate cardiac model on which to conduct disease modeling, drug testing and other research.
One new way to study the heart is through the use of miniature microfluidic chips. These silicone-based rectangular pieces are about the size of a soda can tab and have tiny, specially designed channels where cells are deposited. The cells organize and grow into tissues that mimic organs at a much smaller scale.
The tissue in the chip responds to pharmaceuticals and diseases just like a human heart would respond in the body, allowing researchers to closely observe the responses without the potential for harm.
Mehdi Nikkhah, an associate professor of biomedical engineering in the Ira A. Fulton Schools of Engineering at Arizona State University, and biomedical engineering doctoral student Jaimeson “Jaime” Veldhuizen took on the challenge of designing and validating a new heart-on-a-chip platform. Their innovative work was recently published in the research journal Biomaterials.
Their chip improves upon other engineered cardiac tissue platforms to provide more control and accuracy to study drug toxicity, diseases and treatments.
Advancing the heart-on-a-chip model
Nikkhah and his lab group have been very active in the development of organ and disease tissue-on-a-chip platforms, including neurovascular tissue as well as breast and brain tumors.
“Development of these tissue-on-chip models has generated a rich amount of knowledge and technical expertise in our lab in pertinent areas such as design and fabrication of the microfluidic platforms, selection of proper hydrogel biomaterials, multiculture of primary and stem cells, surface chemistry and so forth,” Nikkhah said. “This generated knowledge, expertise and toolkits have laid a solid foundation for our work in successful engineering of target tissue-on-chip models.”
Organ-on-a-chip technologies have been developed in a variety of structures and cell configurations for some time now. Developing heart patches for therapeutic use has been common, and other engineered tissues in different platforms have been used to research them.
Some investigators use a 2D sheet of tissue, but this doesn't mirror the three dimensions of a human heart. Others employ posts around which engineered tissue is wrapped — a format that is good for measuring contractions of the heart muscle.
One drawback to these types of engineered tissues and other chip platforms is they typically use only cardiomyocytes, which are the cells that make up the heart muscle. By only using one cell type, or monoculture, the studies are limited because they don’t consider other important cell types that make up the wall of the heart.
To better mimic the heart as a whole, Veldhuizen and Nikkhah created a unique design that differs from others in key ways. Their model is the first 3D microfluidic heart-on-a-chip that uses both cardiomyocyte muscle cells and fibroblast connective cells. The chip’s tissue channels also have microposts around which the tissues grow in a more organized structure.
Getting to the heart of the problem
If you look at the wall of a heart muscle through a microscope, you will see that the tissue, called the myocardium, is composed of parallel, aligned fibers that enable regular heartbeats.
Veldhuizen and Nikkhah mimic this structure on a chip platform with elliptical microposts — a unique design for organ-on-a-chip platforms.
“The importance of these posts is their ability to affect the surrounding 3D tissue, causing it to align around the posts,” Veldhuizen said. “We found that these posts can align heart tissue in a similar fashion (to natural myocardial tissue), which not only enhances the structure of the tissue, but also its function, making it a better heart-on-a-chip model.”
Another way to better represent a real heart is to use more than just muscle cells. Cardiomyocytes enable the heart muscle to beat throughout a person’s life, but they need their support structures, the fibroblast connective cells, to reliably function.
Creating these cells is a challenging task for researchers — and Veldhuizen had to do it three times with different stem cell types. In the final stage of the research, Veldhuizen used human pluripotent stem cells to create cardiomyocytes. Pluripotent stem cells are expensive and hard-to-produce, but they are very beneficial as they can turn into any type of organ cell. But turning them into the right type of organ cell is a researcher's biggest challenge.
Typically, researchers use specific stimuli or reagents to make human pluripotent stem cells “differentiate” themselves into the type of cells they want. But sometimes the stem cells spontaneously start on their journey to become something the researchers don’t want, like kidney cells. In Veldhuizen’s work, she’d sometimes see spontaneous differentiation of the stem cells, where they'd become some mixture of cells instead of the heart cells they needed to use in their model.
Even when Veldhuizen got the cardiomyocyte cells she wanted, another obstacle she encountered was their immaturity. When first differentiated, stem cells reflect tissue cells in an embryonic or fetal state, not how typical adult cells look and behave.
“The ability to mature human pluripotent stem cell cardiomyocytes is a major obstacle in the field of heart tissue modeling,” Veldhuizen said. “The differences between the fetal and the mature or adult types span cell size, shape, metabolism, gene expression, calcium handling and contractile patterns. Therefore it is important to mature these cells to model the adult human heart in a lab, and to translate any biological or pharmaceutical findings into clinical relevance.”
Veldhuizen found that by both co-culturing the muscle cells with supporting fibroblast cells among a 3D hydrogel and causing them to align with the microposts, her research team’s heart-on-a-chip model enhances the maturity level of these heart cells, so they’re more suitable for in vitro heart studies.
A multidisciplinary team tackles a complex organ
Just as the human body uses a team of specialized organs to function, complex health technology requires an interdisciplinary group of researchers to get results.
Veldhuizen and Nikkhah worked with stem cell biology expert David Brafman, an assistant professor of biomedical engineering and faculty member of the ASU-Banner Neurodegenerative Disease Research Center, as well as recent bioengineering and biomedical engineering doctoral degree graduate Joshua Cutts.
Brafman and Cutts provided guidance on the foundations of differentiating commercial stem cell lines into heart tissue, and access to a quantitative polymerase chain reaction machine in their lab, which is used for gene expression analysis.
To ensure their work is clinically relevant, the team consulted with Dr. Raymond Migrino, a cardiologist and cardiovascular biology expert from the Phoenix Veterans Affairs Health Care System and the University of Arizona.
Working together with experts in these areas allowed Veldhuizen and other members of the Nikkhah Lab to design a chip that resulted in the best functional model of a heart for a range of important uses.
A new way to study heart diseases, treatments and drug effects
Now that Veldhuizen and Nikkhah have validated the technology, it can be used as a platform to model the progression and treatment of cardiovascular diseases.
This is an important platform for testing pharmaceutical effects on the heart. Many drug discovery approaches fail in preclinical and clinical trials because the conventional assays used in earlier tests do not sufficiently mimic the human heart. As well, researchers can bypass animal models that do not accurately reflect human physiology.
By introducing drugs to tissue on the chip, researchers can observe toxicity and side effects that would negatively affect the heart. For example, chemotherapy often leads to cardiac toxicity, but no one knows why. Researchers can study this phenomenon with Veldhuizen and Nikkhah’s new platform.
In addition to drug experiments, researchers can introduce “insults” such as oxygen deprivation for a heart attack or high glucose to simulate diabetes-related heart disease as a means to study common ailments, or to discover causes of diseases that are currently unknown.
Finally, since it has been validated for use with stem cells from adult humans — the pluripotent stem cells — the platform can use a particular individual’s cells to study their own genetic diseases. In the future, the individual’s care team can safely find the most effective treatments by experimenting on the chip and not the person’s heart.
“We can study disease manifestations, mechanisms for how it progresses or different kinds of treatments,” Veldhuizen said.
More Science and technology
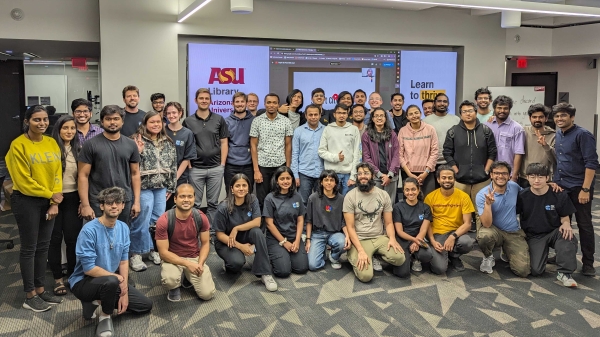
SpaceHACK highlights student solutions to environmental challenges, digital divide
By Adrianna Nine About 250 students from around the world convened online and at Arizona State University on March 22 for the…
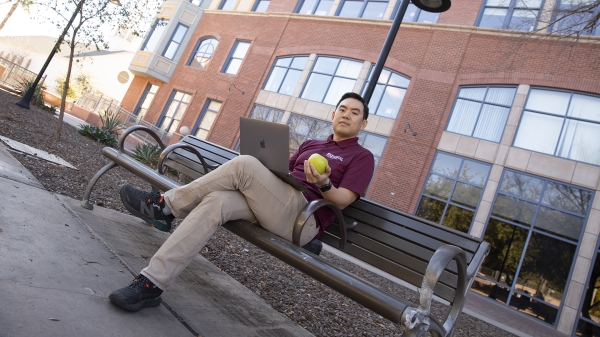
New AI for a new era of discovery
As the legend goes, in 1665, Sir Isaac Newton sat in his garden at Woolsthorpe Manor in England and looked on as a lone apple…
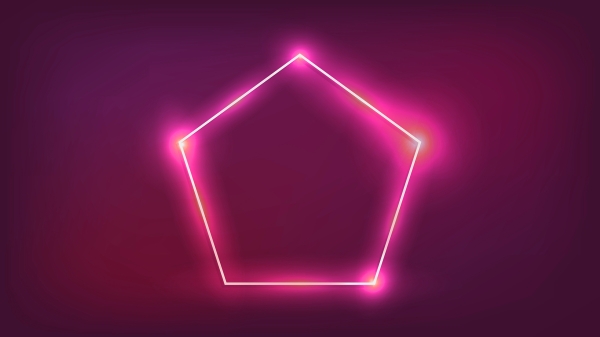
ASU receives 3 awards for research critical to national security
Three researchers in the Ira A. Fulton Schools of Engineering at Arizona State University have received grant awards under the …