The art of letting go: Researchers track progress of separations field in spearheading diagnostics
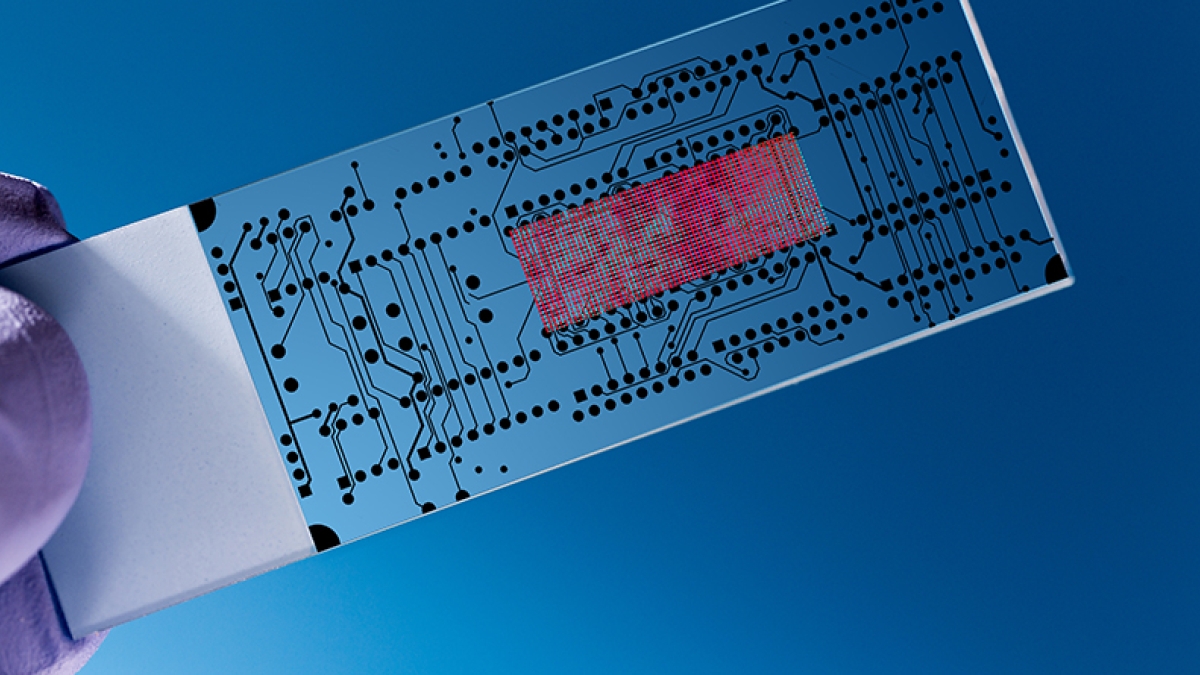
Just as a cotton gin separates cotton fibers from seeds, separation methods for complex biological samples are often required to ferret out targets of interest for researchers and physicians.
Just as a cotton gin separates cotton fibers from seeds, separation methods for complex biological samples are often required to ferret out targets of interest for researchers and physicians. Diagnostic tests may require the separation of certain classes of cells from blood and specific proteins or DNA markers for disease from plasma.
This field has come a long way, and there are a wide variety of methods employed to separate biomarkers or bioanalytes from a sample. Part art, part science, such separation techniques are becoming ever more sophisticated. However, many large-scale methods are time- and resource-intensive. So how do researchers sidestep this? They take everything and make it smaller.
That’s the idea behind “microfluidic” (or nanofluidic) devices. These inventions can accomplish the same task of separating cells and biomarkers used in conventional benchtop separation techniques, but reduce the resources needed and thus the cost. Microfluidic devices take many forms, depending on specific need. Some are modeled like human tissue, and others work by exploiting electric fields or channels that divide particles by charge or size.
Functioning much like small factories, all the internal mechanisms are pre-set to sort a given sample’s components, and because all the materials needed for the separation are confined within the device, fewer specialized skills are required to use them.
“Twenty years ago, there were more specific groups that had to use (large-scale) facilities, and they would understand how to build these devices, so the community was smaller,” said Alexandra Ros, faculty member in the Biodesign Center for Applied Structural Discovery and associate professor in the School for Molecular Sciences. “Now, you can purchase devices — there are no specialized skills required to fabricate them anymore.”
One method of generating these microfluidic devices includes 3D printing. As many know, 3D printers are capable of making objects ranging anywhere from jewelry to the metal parts used in assembling airplanes. But recently, 3D printing technology has been exploited for making these micro- and nanofluidic devices and all their components, eliminating the need for human labor and specialty facilities.
“Think about the 3D printer — when you have the device, you switch it on and feed it a drawing, which is different from having to be in a clean room and building it yourself,” Ros said.
In a review paper published in Annual Review of Analytical Chemistry, Ros — along with first author and postdoc Mukul Sonker— highlighted recent progress in the field. They discuss the advantages and disadvantages of separations in micro- and nanofluidic devices versus their macrocounterparts and review the separation techniques employed within these devices.
“The aim was to summarize separation phenomena that can only be achieved at micro- to the nanoscale,” Sonker said. “We have focused on some unique techniques that can only be realized at these scales and are novel in terms of their separation aspects.”
These techniques include dielectrophoresis and electrophoresis methods. In dielectrophoresis, a biological particle is subjected to a nonuniform electric field exerting a dielectrophoretic force, leading to migration. For biomedical purposes, dielectrophoresis is used to characterize cell types.
In electrophoresis, particles, upon being subjected to a uniform electric field, separate based on charge. This is a common technology for separating nucleic acids or proteins from a human sample.
The paper additionally discusses two size-based separation methods: lateral displacement separation and ratchet mechanisms. In these phenomena, there are obstacles placed within the device. As the particles travel through a channel, these obstacles prompt the particles to arrange themselves by size. These can be used with or without an electric field present and are typically applied to separate cell types of different sizes, such as tumor cells, red blood cells and white blood cells.
Lastly, in entropic trapping, biomolecules (namely DNA) are arranged by level of entropy, which in this case gauges the number of arrangements that molecule can take. There are shallow and deep channels that trap biomolecules based on their entropy level, which often also corresponds to size.
The review paper provides ample background on each technique, offering a useful resource for anyone within or outside the field.
“This review gives a very detailed update about these micro- to nano-scale separation techniques along with their theoretical background and is a good resource for new members in the field to learn about what has been reported already and where the current research is heading,” Sonker said.
The accessibility and resourcefulness of such technology is a testament to the rampant interest in the field.
“The field of microfluidics is just exploding, and there are so many different applications people find useful,” Ros said.
More Science and technology
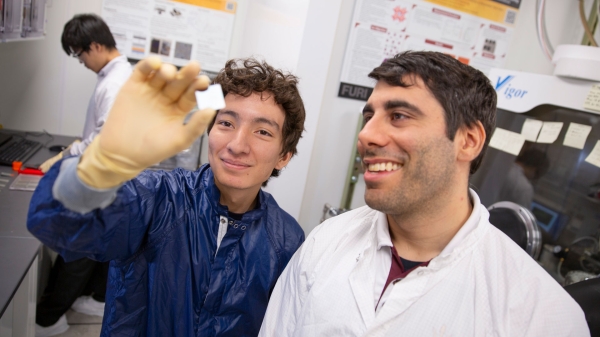
ASU student researchers get early, hands-on experience in engineering research
Using computer science to aid endangered species reintroduction, enhance software engineering education and improve semiconductor…

ASU professor honored with prestigious award for being a cybersecurity trailblazer
At first, he thought it was a drill.On Sept. 11, 2001, Gail-Joon Ahn sat in a conference room in Fort Meade, Maryland.…

Training stellar students to secure semiconductors
In the wetlands of King’s Bay, Georgia, the sail of a nuclear-powered Trident II Submarine laden with sophisticated computer…