New study sheds 'far-red' light on the mysteries of photosynthesis

Some species of cyanobacteria can perform a surprising feat. When deprived of direct sunlight, they are able to use long-wavelength light to carry out photosynthesis. Such organisms acclimate to the far-red light by switching from their normal form of chlorophyl — known as chlorophyll a — to an alternate form, chlorophyll f. This allows absorption of light with wavelengths above 680nm. The behavior highlights the plasticity of processes in nature and may one day be harnessed to engineer plants and other photosynthesizers that can be grown under shaded conditions. Graphic by Shireen Dooling for the Biodesign Institute at ASU
Some 3 billion years ago, tiny organisms known as cyanobacteria helped create an oxygen-rich atmosphere on Earth. Their activities, which continue to the present day, provide an essential ingredient for all advanced life. Such organisms convert radiant sunlight into useable energy through photosynthesis, yet scientists are still sketchy on the details of this vital process.
In a new study, Penn State joins colleagues from Arizona State University’s Biodesign Institute. Their investigations of photosynthesis demonstrate that certain types of cyanobacteria are able to acclimate to faint, long-wavelength light (known as far-red light) not normally captured by plants and other species of cyanobacteria. This remarkable ability gives these living forms an adaptive edge in environments where direct sunlight is limited.
Many species of cyanobacteria are common in both aquatic and terrestrial environments. When they find themselves obscured by other organisms, for example, beneath a pond surface or on a forest floor, some are able to acclimate, harvesting the weaker sunlight filtering down to them and using it to drive photosynthesis.
The talent of cyanobacteria for modifying their own architecture and gathering far red light from their environment highlights the remarkable adaptive and acclimation mechanisms present throughout nature. This newly discovered ability in some microorganisms could one day be harnessed to engineer crops that thrive under shaded conditions and may inspire innovations in sustainable energy.
The group’s findings appear in the current issue of the journal Science Advances.
Atmospheric assistants
Cyanobacteria are a diverse group of photosynthetic bacteria, flourishing in moist soils and aquatic environments, living freely or in symbiotic relationships with other life forms. Chris Gisriel, formerly a researcher at ASU’s Biodesign Center for Applied Structural Discovery and currently a postdoctoral associate at Yale, is the lead author of the new study. He emphasizes the critical importance of these microbes for humans and all other life.
“Cyanobacteria make a huge contribution to the total amount of photosynthesis that's occuring on Earth, which means, the oxygen that we breathe,” Gisriel said.
It also produces the primary biomaterials upon which all life on Earth depends.
Indeed, cyanobacteria represent about 50% of all earthly photosynthetic activity or primary production — supplying an oxygen-rich atmosphere conducive to life and a rich food supply for aquatic life. Cyanobacteria are so plentiful and ubiquitous across the earth that they reign above all existing plant life in terms of generating oxygen, making them the most important primary producers on the planet. Their ability to thrive in virtually any environmental niche and their simple growth requirements allow them to flourish in astonishing abundance.
“As a postdoctoral researcher in the 1970s, I studied a similar type of acclimation of a different part of the photosynthetic apparatus in cyanobacteria, a process known as complementary chromatic acclimation, which affects light-harvesting proteins that do not contain chlorophyll,” said Don Bryant, Ernest C. Pollard Professor at Penn State. “Forty years later, one of my students serendipitiously discovers a chlorophyll-based version of a similar phenomenon, and I am back to the future studying something I had worked on with a much more limited biochemical toolkit than my lab has now. What comes around goes around even in science sometimes.”
The current study focuses on Fischerella thermalis, a terrestrial cyanobacterium that has been used in the past as a model organism for the study of photosynthesis. When such cyanobacteria are deprived of the white light most conducive to their growth and photosynthetic activities, they shift gears in order to process far-red light.
The secret to their success, as Gisriel explains, is in their light-harvesting apparatus, specifically, proteins containing chlorophyll.
“Chlorophyll is what makes plants green. It’s also what does the light harvesting — it’s the antenna,” Gisriel said. When chlorophylls in a cyanobacterium’s protein structure absorb light, they convert it into the metabolic driving source powering the cell.
The complex machinery of photosynthesis is carried out in two primary reaction centers, known as photosystem I and photosystem II, (PSI and PSII). The current study focuses on PSI, teasing out the alterations in F. thermalis that permit it to access and use far red-light for photosynthesis.
How do they do it?
“Understanding how photosynthesis has evolved in different flavors and in different environments is what we're really interested in,” Gisriel said. “The ability to absorb far-red light is what this paper is about.” Like all species of cyanobacteria, F. thermlis is rich in chlorophyll.
But not all molecules of chlorophyll are created equal. In the case of F. thermalis, its usual complement of chlorophyll, known as chlorophyll a, is partially replaced under far-red light conditions with a closely related yet chemically distinct form of the molecule, known as chlorophyll f. It is this alternate form of chlorophyll that enables F. thermalis to harvest and use far-red light to continue photosynthetic activities. By synthesizing and incorporating around 8% chlorophyll f into their photosystem I (PSI) complexes, F. thermalis is able to carry out photosynthesis using far-red light of up to nearly 800 nm.
“If you put these organisms in white light, they only use chlorophyll a and they're just like all the other cyanobacteria,” Gisriel said. “But if you move them to the shade, where they have more of this lower energy, far-red light, they actually switch out some of the chlorophyll a's for chlorophyll f, and that allows them to absorb far-red light. That’s a testament to the plasticity of photosynthesis — it can adapt to many environments, which I think is a pretty incredible mechanism.” This process is controlled by a protein that senses the incident light wavelength, and activates the production of the modified photosynthetic apparatus only when far-red light is predominant over visible light.
Research suggests that perhaps 25% of all cyanobacteria can access and use far-red light for photosynthesis. This would imply that a significant portion of net primary production on Earth is a direct result of this unusual adaptation.
Looking deeper
Examining the structural makeup of protein complexes like PSI requires researchers to peer into natural phenomena occurring at the nanometer scale (i.e., billionths of a meter). One of two methods are usually used. The first, known as X-ray crystallography, produces detailed structural information by striking crystalized samples with high intensity X-ray light, producing a series of diffraction patterns that can be computer-assembled into high resolution images.
The second method and the one applied in the current study, is a powerful new microscopy technique, known as Cryo-EM (for cryogenic electron microscopy). Here, instead of crystallizing samples for study, they are cooled to very low temperatures and embedded in ice. The method allows for structural determination without the need for crystallization and can determine biomolecular structures with near-atomic scale resolution. The technique has proven so powerful, its invention was honored with the 2017 Nobel Prize in Chemistry.
Cryo-EM involves gathering thousands of images of sample particles in various orientations and using computer algorithms to re-assemble the images into a detailed, three-dimensional composite, known as a density map. Using the method, the researchers were able to solve the structure of PSI, revealing the locations of chlorophyll f molecules present in F. thermalis responsible for far-red light acclimation. Cryo-EM is a particularly powerful technology for solving the structure of large, complex proteins and complexes like PSI, which have been challenging targets for X-ray crystallography.
Petra Fromme, director of the Biodesign Center for Applied Structural Discovery and co-author of the new study elaborates: “Isolating PSI — a huge million-atom complex — and keeping it stable until you can determine its structure is very challenging. It took us over a decade to solve the first ever PSI structure using powerful X-rays two decades ago. With Chris’s leadership, we were able to solve the structure of PSI from a completely new organism in just one year using ASU’s state-of-the-art Cryo-EM, which is quite remarkable.”
Red dawn
Scientists would like to know more about the evolutionary underpinnings of far-red light exploitation by photosynthetic organisms. Presumably, when population numbers caused cyanobacteria to be in fierce competition for the visible light component of direct sunlight, strong selective pressure caused some to find an alternate means of carrying out photosynthetic operations essential to their survival. For this reason, it is believed that such organisms first developed chlorophyll a and that chlorophyll f likely evolved later, though no one is sure.
The work offers only tentative steps in the direction of understanding far-red light photosynthesis. Nevertheless, the findings suggest exciting possibilities for future applications. Crops could potentially be tweaked to control their light absorption properties depending on ambient light conditions. Perhaps two crops could be grown in conjunction, with shorter crops like alfalfa extracting far-red light from their shaded location beneath a taller crop like corn. Such an arrangement would allow double the crop yield per unit area. The process is also not limited to crops but could theoretically be applied to any useful photosynthetic organisms.
Further afield, a better understanding of far-red light acclimation in cyanobacteria could also inform a new generation of synthetic light-harvesting technologies like photovoltaics, potentially increasing their versatility under varying sunlight conditions.
ASU connection
The ASU team included faculty working at the Biodesign Center for Applied Structural Discovery and the School of Molecular Sciences including Center Director Petra Fromme. The researchers include first author Chris Gisriel, with support from Shangji Zhang and Dewight Williams. Gisriel conducted these experiments while a postdoctoral associate in the Biodesign Center for Applied Structural Discovery at ASU and since has joined the group of Gary Brudvig at Yale University as a postdoctoral associate.
Other authors on the paper include Gaozhong Shen, associate research professor in biochemistry and molecular biology; Vasily Kurashov, assistant research professor in biochemistry and molecular biology; and John Golbeck, professor of biochemistry and biophysics and of chemistry, all at Penn State. Author Ming-Yang Ho was a graduate student in biochemistry and molecular biology at Penn State when he participated in the research and is now an assistant professor of life science at National Taiwan University.
This work was supported by the National Science Foundation and the Biodesign Center for Applied Structural Discovery at Arizona State University. Some of this research was also conducted under the auspices of the Photosynthetic Antenna Research Center, an Energy Frontier Research Center funded by the Department of Energy. The work made use of the FEI Titan Krios (NSF 1531991) at Arizona State University.
More Science and technology
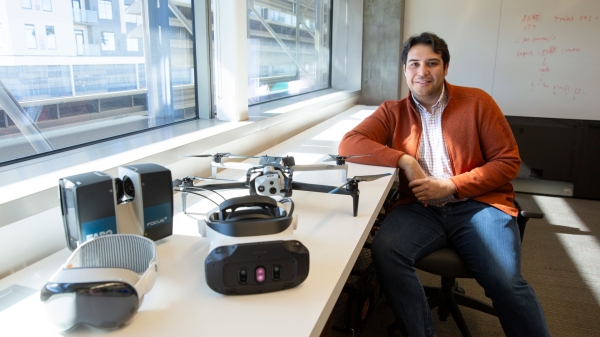
Teaching construction realities with virtual environments
Visiting a construction site is a valuable learning opportunity for students who want to one day work in the industry.…
ASU, Mexico partner to build next generation of chipmakers, drive semiconductor innovation
Thousands of college students in Mexico will soon have the opportunity to enroll in Arizona State University’s new, free online…

ASU, St. Mary’s Food Bank partner to tackle food insecurity in Arizona
Arizona State University and St. Mary’s Food Bank (SMFB) have joined forces to create an interactive data dashboard that tracks…