New electrochemical platform paves the way for advanced portable diagnostic tools
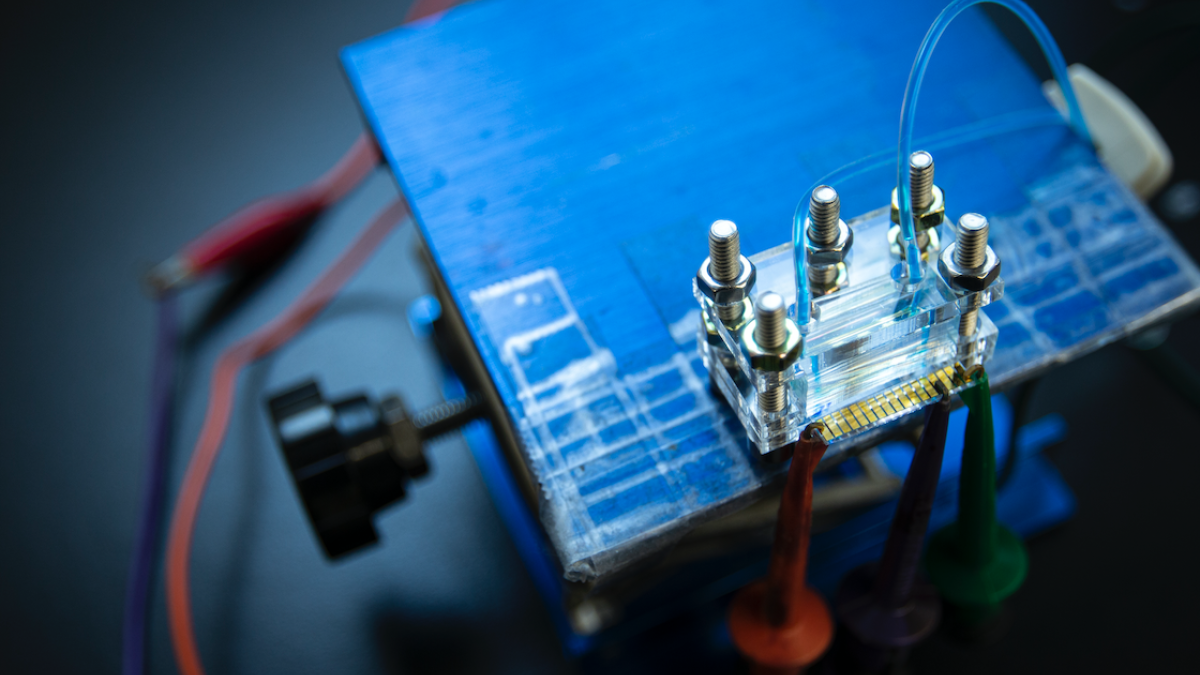
The direct gene-circuit-to-electrode interface unites biology’s ability to sense with the memory and decision-making capabilities of electronic systems. Photo by Steven Southon, University of Toronto
Scientists at Arizona State University and the University of Toronto have developed the first direct gene-circuit-to-electrode interface by combining cell-free synthetic biology with state-of-the-art nanostructured electrodes. Long inspired by concepts from the field of electronics, with its circuits and logic gates, synthetic biologists have sought to reprogram biological systems to carry out artificial functions for medical, environmental and pharmaceutical applications. This new work moves the field of synthetic biology toward biohybrid systems that can take advantage of benefits from each discipline.
“This is the first example of a gene circuit being directly coupled to electrodes and is an exciting tool for the conversion of biological information into an electronic signal,” said Keith Pardee, assistant professor in the Department of Pharmaceutical Sciences at the University of Toronto's Leslie Dan Faculty of Pharmacy.
Study results were published today in Nature Chemistry. The interdisciplinary effort to create the new system brought together expertise in cell-free synthetic biology from the Pardee lab and electrochemistry from the Kelley lab at the University of Toronoto and sensor design from the Green lab in the Biodesign Center for Molecular Design and Biomimetics at ASU.
“This new system enables us to detect many different signals simultaneously, which is essential for diagnostics and monitoring systems,” said co-author Alexander A. Green, researcher at the Biodesign Institute at the Arizona State University and assistant professor in the School of Molecular Sciences. “The electronic output means that in the future it can be readily interfaced with technologies like smartphones and distributed sensing arrays that could be brought directly to a patient’s bedside.”
Pardee, whose research group specializes in developing cell-free diagnostic technologies that can be used safely outside the lab, and Green, whose research group engineers molecular sensors, received widespread attention in 2016 when they and collaborators released a platform for the rapid, portable and low-cost detection of the Zika virus using paper-based synthetic gene networks.
“In our previous work detecting the Zika virus, we showed how you could embed the cellular machinery on a piece of paper, a nonbiological environment, to detect pathogens at very low cost,” Green said. “With this new work, we’re showing how you can connect the same cellular machinery to an electronic interface and detect multiple independent signals at the same time.”
“With these hybrid biological-electronic systems we have the chance to take full advantage of biology’s ability to sense along with the capacity of electronic systems to process huge amounts of information, which should enable us to make more advanced and broadly useful diagnostics going forward,” he said.
Overcoming practical limits of optical signaling
Bringing the capacity to detect the Zika virus outside of the clinic and to the point of need was a crucial step forward, but the approach relied on conventional optical signaling — a change in color to indicate that the virus had been detected. This posed a challenge for practical implementation in countries like Brazil where viruses with similar symptoms require health care providers to screen for several different pathogens in order to correctly identify the source of a patient’s infection.
This highlighted the need for a portable system that could accommodate many sensors in the same diagnostic test, a capability known as multiplexing. The challenge was that multiplexing with color-based signaling is not practical.
“Once you get beyond three color signals, you run out of bandwidth for unambiguous detection. Moving into the electrochemical space gives us significantly more bandwidth for reporting and signalling. We’ve now shown that distinct electrochemical signals can operate in parallel and without crosstalk, which is a much more promising approach for scaling up,” Pardee said.
The new biohybrid system uses nonoptical reporter enzymes contained within 16 microliters of liquid, about a third the size of a water droplet, which pair specifically with micropatterned electrodes hosted on a small chip no more than one inch in length. Within this chip, gene circuit-based sensors monitor for the presence of specific nucleic acid sequences, which, when activated, trigger the production of one of a panel of the reporter enzymes. The enzymes then react with reporter DNA sequences that set off an electrochemical response on the electrode sensor chip.
Detecting antibiotic resistant genes
As a proof of concept, the team applied the new approach to detecting colistin antibiotic resistance genes that have recently been identified in livestock globally and represent a serious threat to the use of the antibiotic as a last resort treatment for infection. Four separate resistance genes were detected, demonstrating the ability of the system to effectively identify and report each gene independently, and also in combination.
For synthetic biologists, this new approach represents a potential technical leap forward. Conventional synthetic biology requires that logic calculations be encoded into the DNA of the gene circuit. This can be painstaking, taking months or years to build complex circuits.
“What makes this combined approach so powerful is that the underlying connectivity of the gene circuit sensor outputs can be reprogrammed at will by simply modifying the code at the level of the software rather than at the level of the DNA which is much more difficult and time consuming,” said Shana Kelley, a university professor in the Department of Pharmaceutical Sciences at teh University of Toronto whose research group specializes in the development of highly sensitive electrochemical sensors. Bringing biology-based sensing together with electronic-based logic, memory and response elements, has the potential to transform medicine, biotech, academic research, food safety, and other practical applications, she said.
A powerful tool kit for the future
“In the future I think we’ll see these systems deployed in the field for diagnostics that detect panels of different pathogens based on the geographic region or the crops being cultivated to combat disease and increase food production,” Green said. “They’ll also be able to interface with smartphones to automatically analyze test results and gain critical information on how a pathogen is spreading over time.”
In Toronto, Pardee and his research group are excited to see where others in the synthetic biology field will take the system. “We’ve essentially created a new set of tools and opened up a new venue for signaling. Synthetic biology applications are limited at the reporting step and this has been a significant challenge. With this new combined approach, we think we can really accelerate the field and its capacity to improve lives.”
Adapted from story by Kate Richards, University of Toronto. Written by Dianne Price.
More Science and technology

NASA launches space telescope to chart the sky and millions of galaxies
California’s Vandenberg Space Force Base was the site for Tuesday’s 8:10 p.m. launch of the NASA SPHEREx mission aboard a SpaceX…

ASU-led Southwest Sustainability Innovation Engine funds 8 promising tech startups
In the startup world, tech companies often fall victim to what’s known as the “valley of death” — the gap between technological…

New study explores the link between diet, blood sugar and cancer risk across species
Researchers have long known that diet exerts a profound influence on health, including the risk of developing cancer. A new study…