Better brain and body electrical measurements
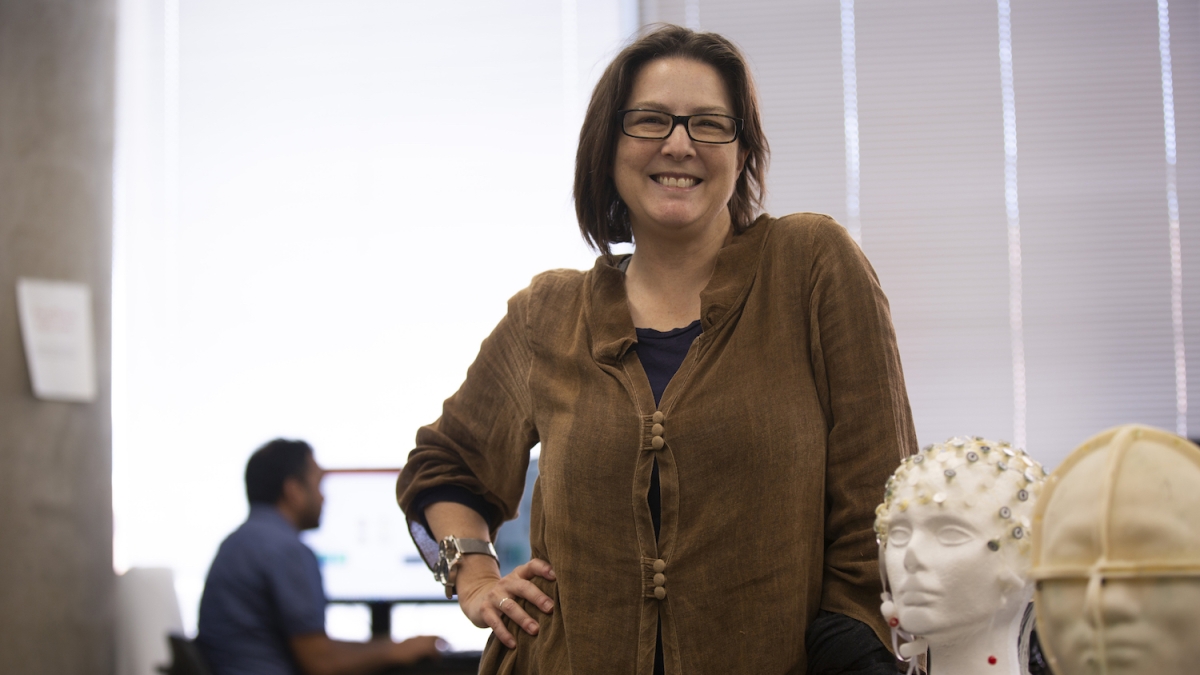
Associate Professor Rosalind Sadleir poses in the Neuro-electricity Laboratory with a model head wearing a series of electrodes used to detect electrical signals in the brain. Sadleir’s ongoing research funded by the National Institutes of Health focuses on accurately measuring the electrical signals of various tissues in the human body, often for the first time. Photo by Erika Gronek/ASU
Your body is bustling with electrical activity. Knowing how cells and tissues conduct electricity is important to understanding readings from medical imaging systems that detect traces of these signals. Distinguishing between normal and unexpected electrical activity in the body is a key part of diagnosing many diseases.
However, measuring the electrical properties of different types of tissue is tricky, and common methods of measurement don’t fully capture what’s happening in the body.
Rosalind Sadleir, an associate professor of biomedical engineering in the Ira A. Fulton Schools of Engineering at Arizona State University, is working to resolve these shortcomings. She’s exploring how new methods and applications of current technologies can better characterize the body’s electrical properties, especially in the brain.
Sadleir’s research is being supported by three National Institutes of Health grants — including one that is part of the Brain Research through Advancing Innovative Neurotechnologies, or the BRAIN Initiative — which fund her work to detect neural activity and map current flow.
“The focus is to figure out the operation of the normal brain and to try to give us some information about the connection between where current is flowing and what parts of the brain are active as a result,” Sadleir said.
Historically, electrical properties have typically been measured with electrodes placed on dissected or biopsied tissues, often from dissected animal tissues or anesthetized animals — an invasive procedure that yields inaccurate results.
“The moment you cut tissue out of somebody, the properties start changing immediately,” said Sadleir, who leads the Neuro-electricity Laboratory where researchers are working to advance the understanding of electrical activity and properties of the brain.
The last major publication of this type of data was released in 1996, with relatively few updates since then. However, many models of tissue electrical properties use this outdated, possibly inaccurate data.
Sadleir wants to go back to square one, gathering the baseline data in humans in noninvasive ways to determine the normal electrical conductance values of living tissues. In particular, she wants to measure the low-frequency range of detection because it is the hardest range to capture and the least measured.
Of most interest to researchers are properties in the range of 1 kHz to 20 kHz because those are the frequencies characteristic of the body’s natural processes. For example, the heart beats around 1 Hz, or once per second, and neural activity mostly occurs in the 1 kHz range. Measurement of electrical properties in this low-frequency range therefore aids understanding of the interplay of activity and conduction of signals through the brain.
Bringing MREIT to life
One method her team is using is magnetic resonance electrical impedance tomography, or MREIT. This involves putting electrodes on a person’s body that deliver an electrical current and create a magnetic field. The person is then placed inside an MRI machine that measures the magnetic field. Through this process, Sadleir’s team is able to characterize living tissues’ electrical properties. Because electrical current can also be used as a therapy, the passage of current to different brain tissues can also be measured, potentially allowing mechanisms of action of these methods to be explored.
“The image we see from the MRI data is the direct effect of the current flow through the tissue,” Sadleir said.
This isn’t a new method, but Sadleir’s team is tailoring it to be used with living humans — a key difference from past use that involved simulated tissues or animal tissues. The results will help scientists conducting neuroscience and neuromodulation research (changing the brain’s activity by applying electricity). In these types of research, accurate values are necessary for developing new treatments and new understanding of the brain.
Beyond low-frequency measurements, Sadleir’s team is also developing a spectroscopic technique to help fill this knowledge gap for living human tissue. Knowing the measurements along the range of frequencies helps reveal the biophysics of the tissue, making it possible to get an accurate picture of what’s happening in the body as it interacts with both the body’s own internal signals, and with externally applied therapies.
In electroencephalogram, or EEG, for example, tiny signals that originated deep inside the body are measured on the scalp to help scientists “see” what’s happening in the brain.
“The way signals travel between the place where they originate and your scalp has all to do with the electrical properties of the tissues in between,” Sadleir said. “In order to figure out where the signals are coming from, people have relied on the old conductivity measurements to make their estimations, which means their estimations are affected.”
Going forward, Sadleir is interested in measuring a wider variety of tissues than brain tissues and at a wider range of frequencies. She is particularly interested in skull bone conductivity “because its properties are very difficult to measure using traditional techniques, and they are critical determinants in locating (electrical signal) sources using EEG.”
“There’s been some controversy about what the properties of the skull are, and they’re extremely important — perhaps the most important tissue — for all of these transcranially applied techniques,” Sadleir said.
Sadleir’s published paper outlining her team’s research results thus far was selected as an Editor’s Choice in Magnetic Resonance magazine.
“(The selection) means there’s something in the research that people in the field appreciate,” Sadleir said. “Which is very good because MRI is something I’ve come to only relatively recently in the last five or six years. So, to actually be doing the research directly and have it recognized is really great.”
This fall, she’s joined by a new cohort of magnetic resonance researchers in the School of Biological and Health Systems Engineering, one of the six Fulton Schools, who will bring a “fantastic breadth of diversity of skills and areas of interest” to the faculty.
More Science and technology

Lucy's lasting legacy: Donald Johanson reflects on the discovery of a lifetime
Fifty years ago, in the dusty hills of Hadar, Ethiopia, a young paleoanthropologist, Donald Johanson, discovered what would…

ASU and Deca Technologies selected to lead $100M SHIELD USA project to strengthen U.S. semiconductor packaging capabilities
The National Institute of Standards and Technology — part of the U.S. Department of Commerce — announced today that it plans to…
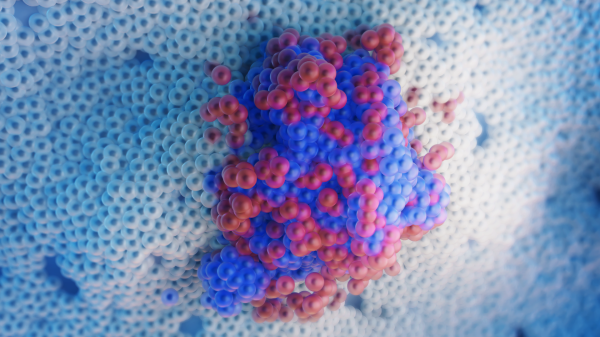
From food crops to cancer clinics: Lessons in extermination resistance
Just as crop-devouring insects evolve to resist pesticides, cancer cells can increase their lethality by developing resistance to…