Biodesign researchers earn grant to investigate virulence in space
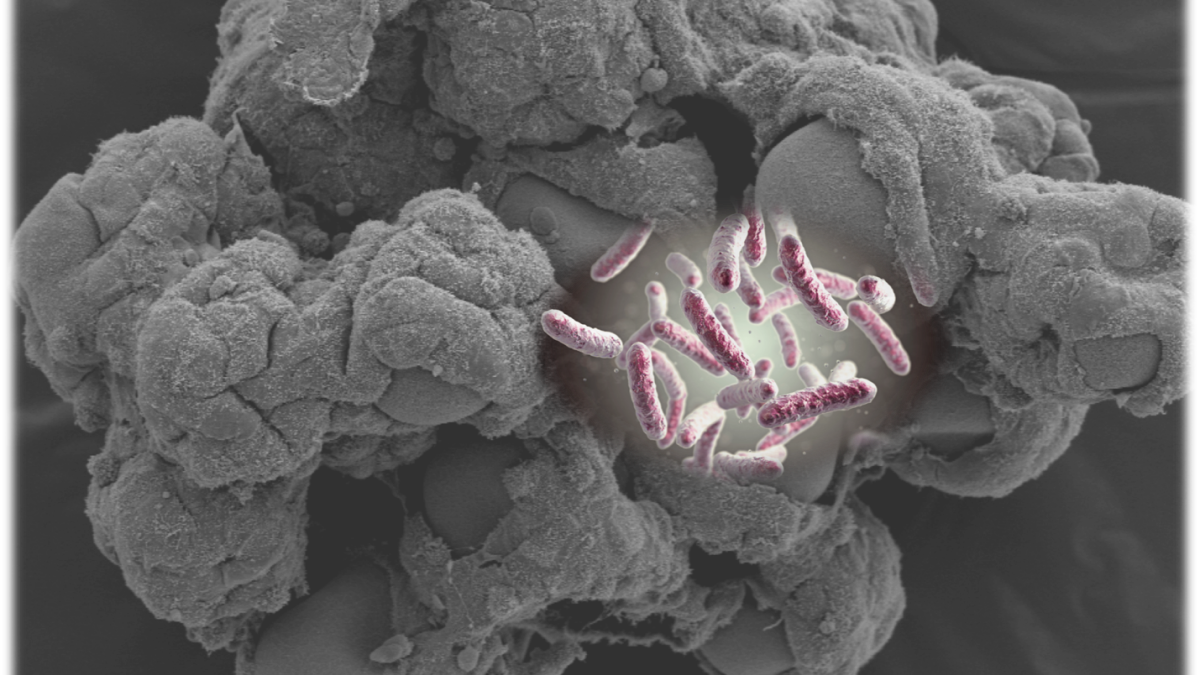
Three-dimensional tissue culture models derived from human cells developed in the Nickerson lab using NASA bioreactor technology bear a striking resemblance to tissues within the body and provide predictive platforms for studying host-pathogen interactions. Bacteria graphic overlaid on the model is from 123rf.com, Image ID: 118061616, copyright Kateryna Kon.
A Biodesign Institute research group has received a three-year, $2.3 million grant from NASA to study and characterize the risks posed by microorganisms to the health of astronauts.
Cheryl Nickerson of the Biodesign Center for Fundamental and Applied Microbiomics and professor at Arizona State University’s School of Life Sciences will be leading the ASU team. Nickerson, a 20-year veteran in the field of infectious disease in microgravity environments, has participated in nine separate NASA spaceflight missions. She partners with ASU Biodesign assistant research professor Jennifer Barrila and Mark Ott, one of NASA’s top microbiologists and the overall project lead at NASA Johnson Space Center.
Microgravity, an extreme environment, can alter cells
The International Space Station (ISS), where the astronauts spend their time, is considered an extreme environment. To explain, the station is in a perpetual state of freefall toward Earth that is only offset by the speed of the station moving at a clip of 17,000 mph. These two opposing forces allow the station to remain in orbit and create the extreme environment known as microgravity. Understanding the response of microbes and tissues in microgravity affords novel insight into how they behave normally. Even more valuable, the extreme environment teaches us how microbes transition to disease states that can be unclear under normal conditions on Earth, where the force of gravity can mask some cellular responses. This work will provide fresh insight into disease processes, including infectious disease mechanisms and immune cell responses to infection.
Studies over the past 50 years have shown that culturing cells (human or microbial) in the spaceflight environment alters them in unexpected ways. For example, certain cells show changes in growth, size, shape, differentiation, antibiotic resistance, biofilm formation and function. In a landmark experiment, Nickerson’s team showed that when cultured in spaceflight, the human foodborne pathogen salmonella significantly altered its gene expression and increased its virulence (ability to cause disease). The team also discovered a specific gene regulator used by salmonella and several other pathogens to regulate their responses to the microgravity environment of spaceflight. These findings are especially concerning regarding infectious disease risks, given that the immune response of astronauts is compromised during spaceflight.
Humans exposed to space travel are found to experience a myriad of issues with their immune systems. These include reduced function and distribution of some immune cells, changes in inflammation and reactivation of latent viruses such as herpes virus. Due to the complexity of virulence studies in microgravity, very few microorganisms have been evaluated in infectious disease studies, and salmonella is currently the only pathogen whose virulence has been shown to change in spaceflight. Accordingly, NASA is concerned with the likelihood of other pathogens that could compromise crew health. Nickerson explains, “The goal of the current study is to conduct comprehensive tests to gain insight into the breadth of other medically-significant ISS microorganisms that have altered virulence and the impact of those changes on the immune response of the host, including astronaut immune cells.”
Analog studies mitigate risk for space travelers
Although NASA takes extreme precautions to avoid crew health risks, infectious disease incidences have been reported on spaceflight missions. Therefore, just as they do with any risk, NASA must gain a proper understanding of any possible outcome of that risk. This avoids potential catastrophes in space. NASA does so with analog testing. Analog testing, grounded here on Earth, uses instrumentation and equipment that can simulate some spaceflight conditions. Nickerson’s team cultivates the pathogenic microbes in bioreactors that can both mimic aspects of the microgravity environment as well as simulate conditions experienced by tissues in our bodies and pathogens that infect those tissues. Specifically, these space analog bioreactors mimic the low fluid force conditions (called fluid shear) encountered by cells in these environments.
Nickerson and her team will be providing a virulence risk profile for a range of pathogens that NASA is concerned about. In the study, the pathogenic microbes will be exposed to a variety of stressors that may be encountered when infecting a host. Nickerson explains, “We are testing the ability for spaceflight analog grown pathogens to resist stresses that your body throws at them during infection, and then we ask can they be effectively killed?” In addition, the ability of these pathogens to infect both 3D tissue culture models (also produced in analog conditions) that contain immune cells and astronaut blood will also be investigated.
“This grant leverages from all of the biomedical work that we have completed with NASA in the past," Nickerson said. "These studies are specifically designed to help chart NASA's mission for what kind of infectious disease health risks might occur during human exploration missions to the moon and Mars."
More Science and technology
2 ASU faculty elected as fellows to National Academy of Inventors
Arizona State University faculty members Bertram Jacobs and Klaus Lackner have been elected as fellows to the National…

Harvesting satellite insights for Maui County farmers
Food sovereignty can refer to having access to culturally significant foods, but Noa Kekuewa Lincoln believes it goes farther…

Google grant creates AI research paths for underserved students
Top tech companies like Google say they are eager to encourage women and members of historically underrepresented groups to…