From a single leaf to a sea of green: The predictive power of leaves for forest ecology

In his poem “Song of Myself,” Walt Whitman wrote: “I believe a leaf of grass is no less than the journey-work of the stars.”
For Arizona State University biologist Benjamin Blonder, the wonder of a single leaf begins upon a closer examination of its veins under the microscope. These fingerprint-like patterns — many unique to a plant species — turn out to have a tremendous potential toward understanding the ecological health of plants, trees and forests.
“If you are an organism, how should you deploy your resources?” asks Blonder, an assistant professor in ASU’s School of Life Sciences. “If you are a plant or animal and you are trying to grow, and you’ve got a certain amount of energy and mass to throw around, are you better off reproducing right away, are you better off growing really big first or are you better off growing really quickly and then reproducing later?”
“In plants, one of the critical axes for that is in their leaves.”
Leaf venation network traits are vital to plant life, from nutrient transport to mechanical support to damage control and pest resistance.
Connecting the patterns found in the veins of leaves drives much of the research focus in Blonder's lab.
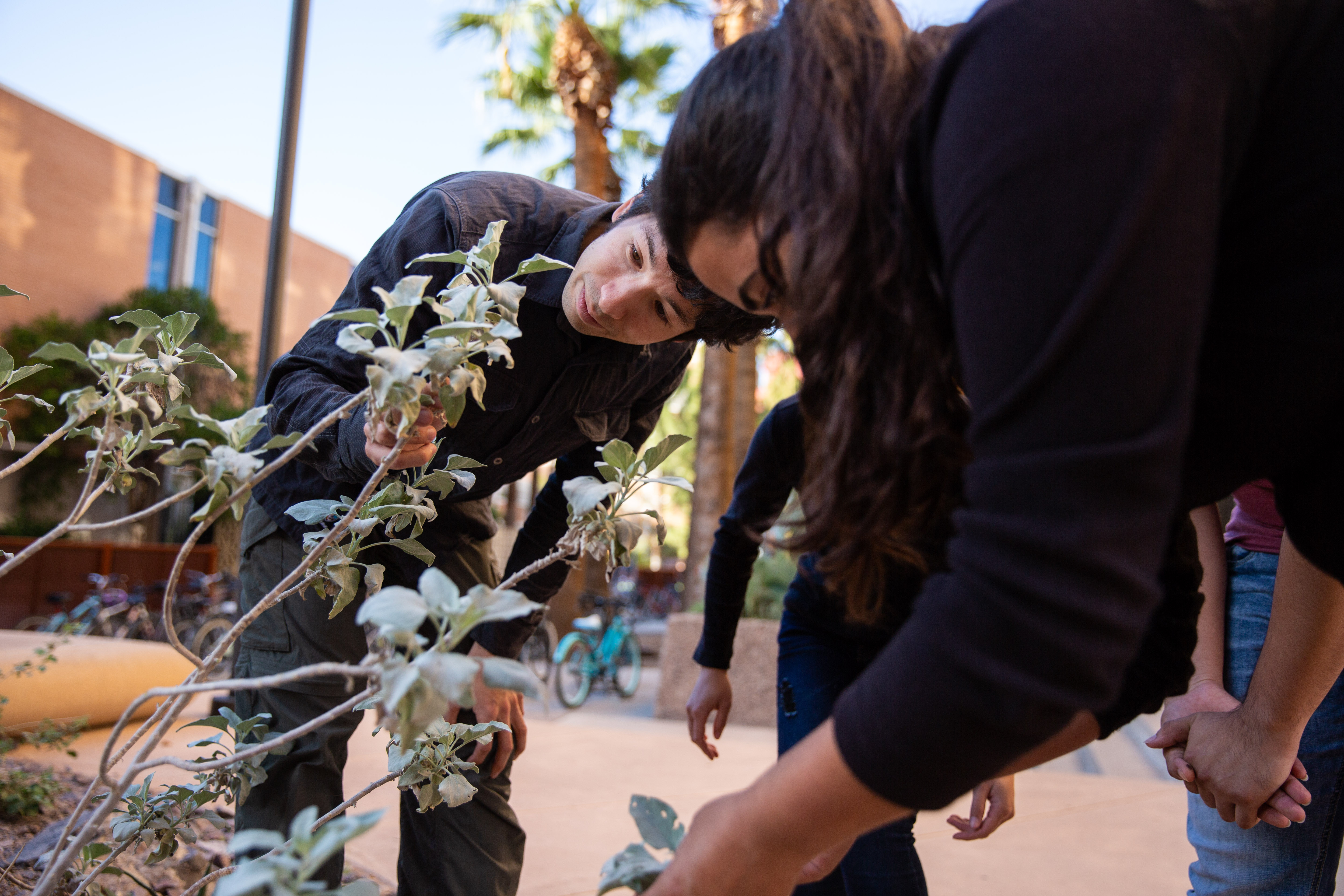
Benjamin Blonder (left) and postdoctoral researcher associate Luiza Teophilo Aparecido examine a brittlebush specimen outside the School of Life Sciences on the Tempe campus Oct. 17. Photo by Deanna Dent/ASU Now
Economies of scale
Leaves are a basic energy unit of biology. But they come at a cost to make. Elements like carbon and nitrogen flow through, costing energy to produce enough nutrients to sustain a single leaf.
“And you can think about a leaf as a vehicle for investment sort of like you would invest in a mutual fund or anything else that could return some interest,” said Blonder. “So, you have some initial outlay of costs to buy the thing which is effectively constructing it, and then photosynthesis returns some resources over time, but it also depreciates. …
“With a plant, you’ve sort of got these trade-offs between resource return rate and initial resource costs and then, the longevity of the resource.”
This “leaf economic spectrum” theory was first developed in a seminal Nature paper by Ian Wright. Blonder, while under the tutelage of doctoral adviser Brian Enquist at the University of Arizona, wanted to explain why it exists. Their hypothesis is that there is a cost and benefit to making a leaf, and when observed repeatedly across the scale of biomes, the trade-offs influence leaf form and function, and the different kinds of vein architectures and patterns seen.
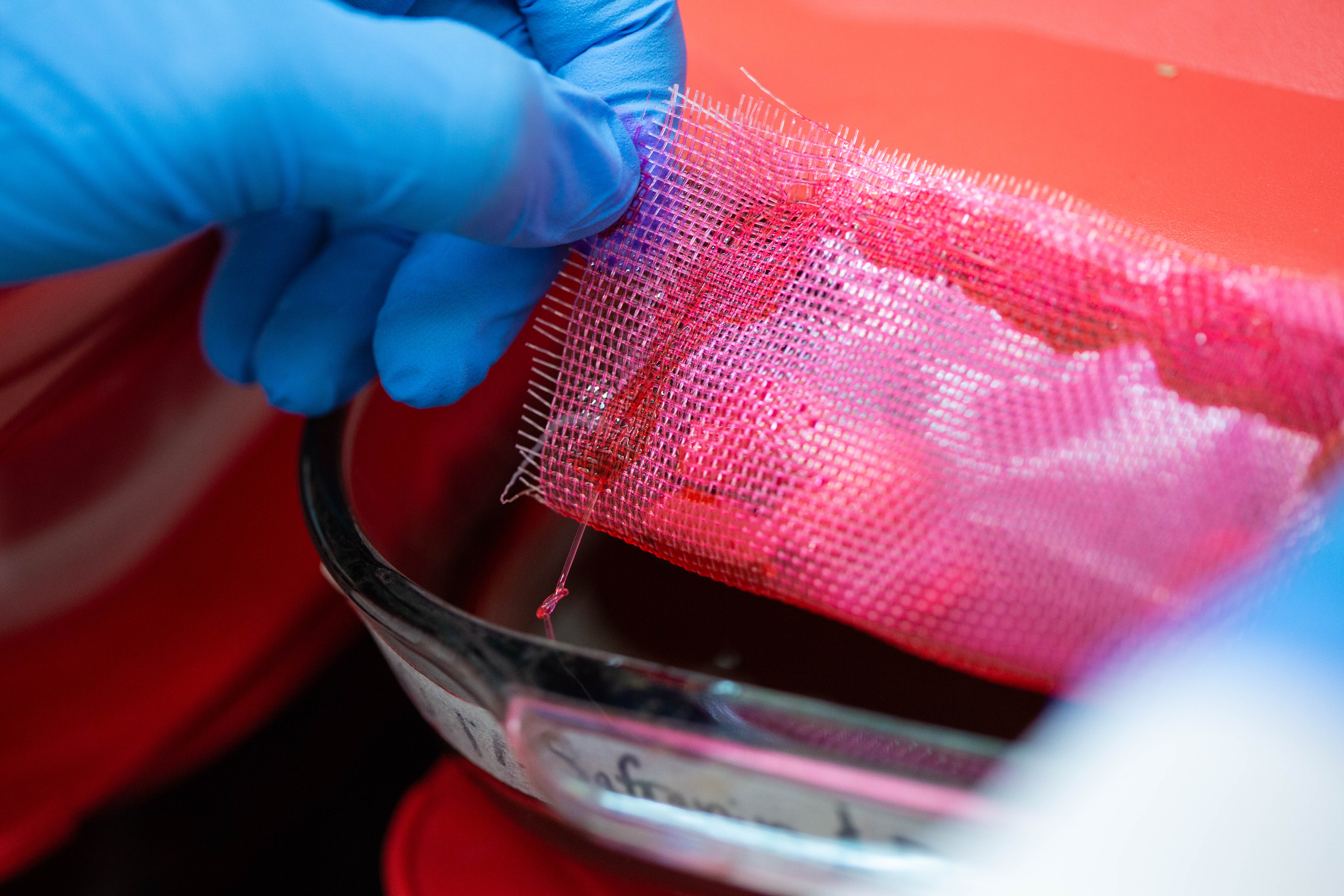
One step in the process of data collection is the dyeing of the leaves in a safranin bath, allowing for more contrast during the imaging process. Photo by Deanna Dent/ASU Now
Under the skin
Postdoctoral researcher Luiza Teophilo Aparecido, a Brazilian tropical forest ecologist, has helped Blonder develop and refine a suite of standardized basic leaf vein measurements.
They start by peeling away the delicate outer layer of leaf skin, the tough lignum. They soak the leaves in a chemical solution to get at the heart of the nourishing waterways.
After an hour, the chemical clearing and a safranin staining reveals a reddish vein network, tied together by a thin, translucent skin of connective tissue. When they examine them under the microscope, there are patterns that jump out, unique and widely varying among different species.
Blonder’s team measures three key leaf vein traits — distance, density and loopiness — and has developed a way to standardize the measurements for these traits. Under the microscope, they take images and use digital-image analysis programs to precisely measure the venation patterns.
“We are usually on a 4X objective for the maximum scale, 500 pixels per millimeter, or 2 microns per pixel when we look at these samples,” said Blonder. “The smallest feature is about 10 microns wide, and the biggest feature, the whole leaf, in the case of some palms, can be up to several meters long.”
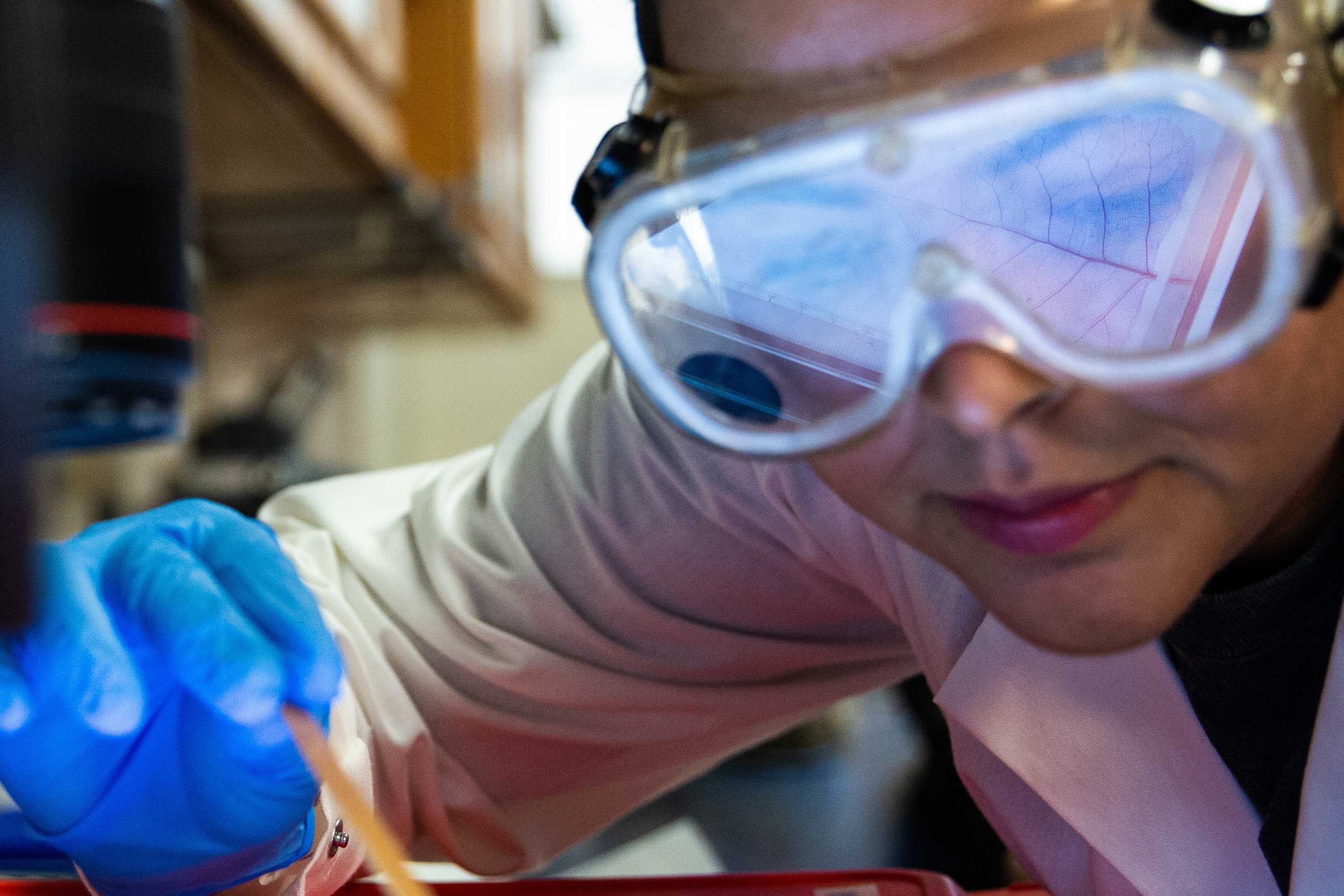
Postdoctoral researcher associate Luiza Teophilo Aparecido examines a cottonwood leaf vein system (reflected in her safety goggles) in the Macrosystems Ecology Lab at the School of Life Sciences on Oct. 17. Photo by Deanna Dent/ASU Now
Turning a new leaf
The pattern of the leaves' vein transportation networks provides a new window into plant form and function.
It can reveal the relationship between the plant water supply and available environmental water supply, and an optimized nutrient and water transport system.
The venation traits mediate different economic strategies across environments. For the longest time, plants were classified based on just form, like maple leaves from oaks. Now, Blonder’s predictive model has the potential to provide insights into leaf form and function across broad climate gradients, as well as broad biogeographic patterns of plant species’ distributions.
“It’s trade-offs in transportation networks within leaves that may force all of these other economic trade-offs downstream,” said Blonder.
His lab has explored this for many plant species, from Arizona and across the globe.
Blonder’s team is continuing their work in Arizona (from the desert to the high-elevation areas known as sky islands) as well as hopscotching their travels from tropical forests to high-diversity regions. This includes Peru in the Andes, the Amazon rainforest, African equatorial forests and temperate forests in Europe and Southeast Asia.
“These (regions have) these very deep branches in the tree of life for plants,” said Blonder. “We kind of want to know, did they all come up with the same kind of solutions or did they not?”
A deeper understanding
In a new project, funded by a recent four-year, million-dollar National Science Foundation award, Blonder is focused on taking on some of the leaf trade-offs and strategies in a more “thoughtful and in-depth way than before.”
Many of these have to do with how a plant interacts with its environment, and the way it defends itself against other organisms.
“There are these defensive functions to plant transportation networks in addition to the simple transport-related ones,” said Blonder. “Most of the mechanical strengths of leaves come from the mechanics of the veins that hold the leaf up. The physical aspects are just as important as the transport part of the system.”
Compare the venation patterns of the lemon tree leaf and the ginkgo tree.
“Imagine you have an insect that takes a little paper-hole-punch-size bite out of each of them. If you take a hole-punch bite out of the ginkgo, everything that is downstream of that hole is dead because you just have these single long strands that are empowering the whole thing. With the lemon leaf on the other hand, there is a very redundant network of loops that says, look, we will just reroute somewhere else.”
Blonder likens it to a city with a single traffic lane. If someone got into an accident, the whole transportation network suddenly becomes gridlock. So humans have built cities with many different avenues leading to the same destination, like the lemon tree leaf's extra loops.
“Now, the interesting thing is that if you have that kind of redundancy, you also have additional costs because creating those loops … does cost more to put in (but) you can make the whole thing mechanically stronger at the same time.”
The NSF funding will also take the often slow and laborious process of leaf vein measurements performed by students and automate it using the latest tools of machine learning, or artificial intelligence.
“What we’ve done is we’ve gotten together with a bunch of people who are doing AI and we’ve taught a computer how to trace these things by themselves,” said Blonder. “So, now all we do is we take the picture, and we go chuck it down a big cluster and go wait an hour, and the computer works really hard and we go have lunch,” he joked. “Suddenly we have data an order of magnitude higher than anyone’s been able to have before.”
The tsunami of new AI-generated data will go a long way toward improving their models.
Video by Ken Fagan/ASU Now
Learning from leaves
At the end of the grant period, Blonder also hopes to push the boundaries of discovery and apply some lessons learned to one day better aid biomimicry applications.
“What we are really doing is creating an empirical knowledge of what transportation networks are like on much bigger and broader special scales than anyone has managed before,” he said. “Now, this is useful for plants, but I also think it is potentially useful for many other biomimicry types of applications.”
In addition to human transportation roads and networks, Blonder mentions electrical power grids, animal environmental adaptations, and even human and animal circulatory networks may be helped by a better understanding of plant resource utilization.
“We would simply like to know how organisms are doing this in nature and when does one of the strategies work well and when do organisms switch over to another strategy? We just don’t know because no one has gotten that data — it’s just hard. So, we are going to try and chase that one down.”
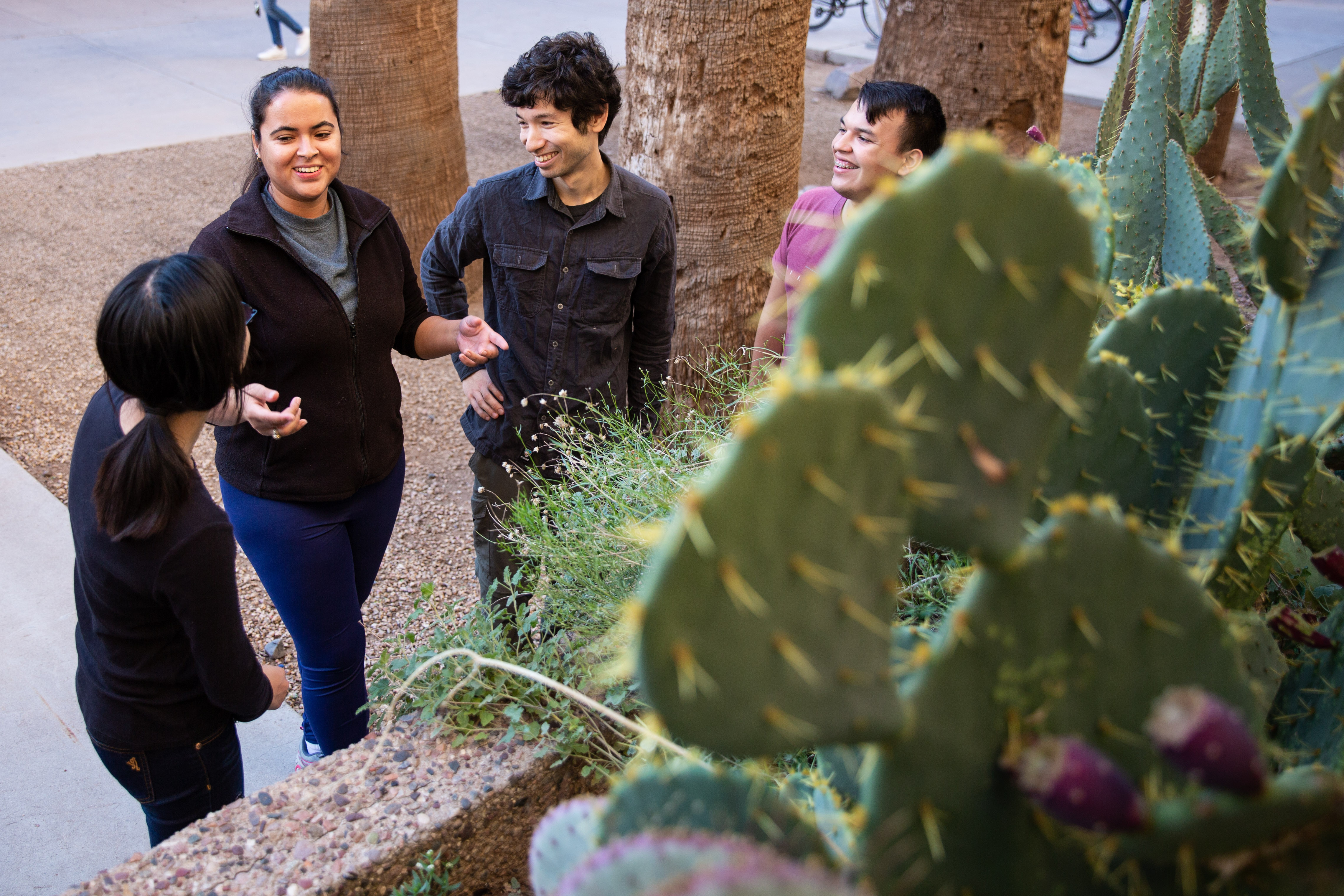
Luiza Teophilo Aparecido and Ben Blonder (center) discuss collection with student researchers Sabrina Woo (left) and Miguel Duarte (right) outside the School of Life Sciences during the fall semester. Photo by Deanna Dent/ASU Now
More Science and technology
Extreme HGTV: Students to learn how to design habitats for living, working in space
Architecture students at Arizona State University already learn how to design spaces for many kinds of environments, and now they can tackle one of the biggest habitat challenges — space architecture…
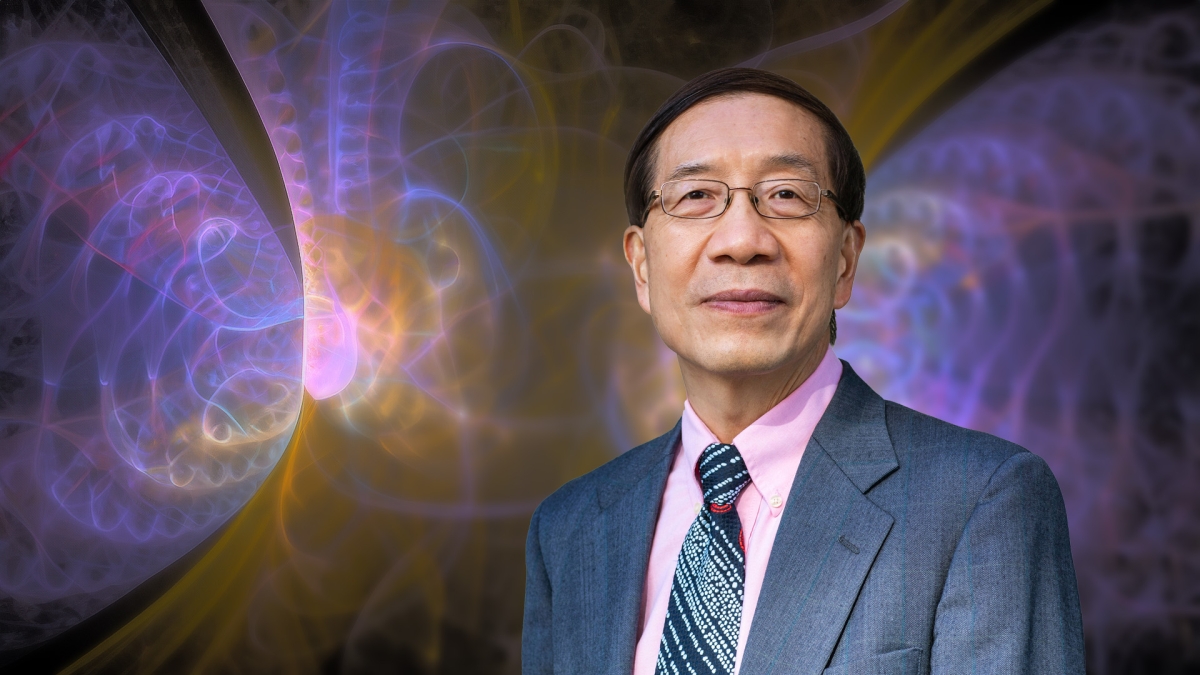
Human brains teach AI new skills
Artificial intelligence, or AI, is rapidly advancing, but it hasn’t yet outpaced human intelligence. Our brains’ capacity for adaptability and imagination has allowed us to overcome challenges and…
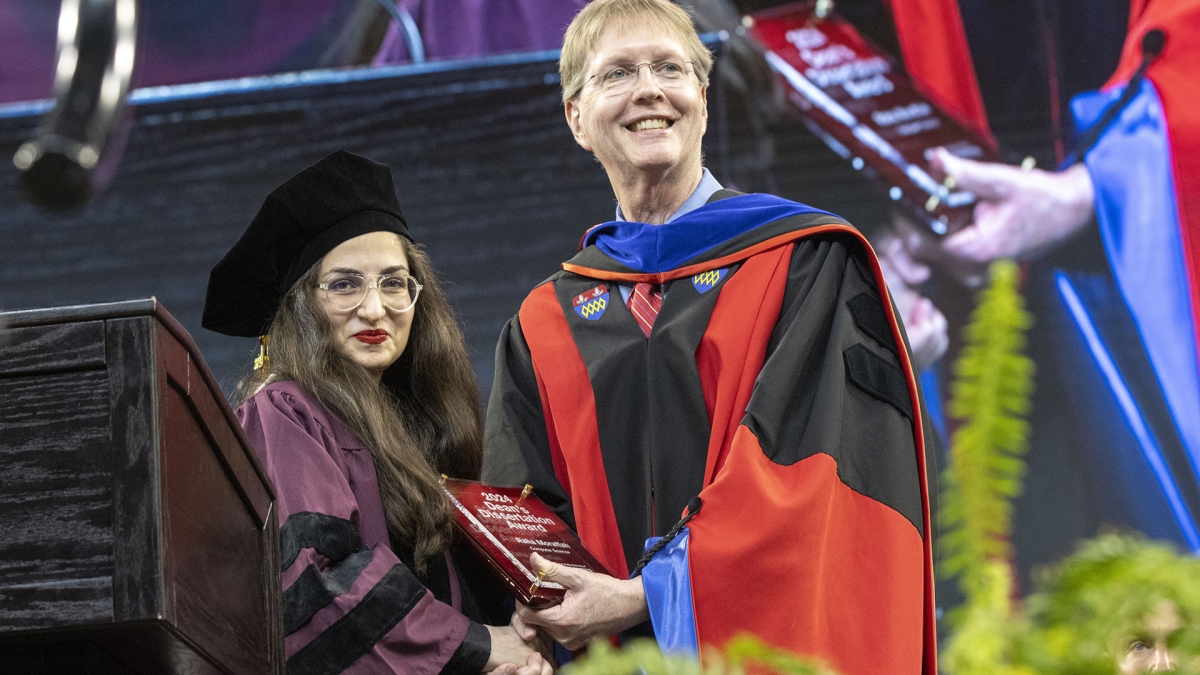
Doctoral students cruise into roles as computer engineering innovators
Raha Moraffah is grateful for her experiences as a doctoral student in the School of Computing and Augmented Intelligence, part of the Ira A. Fulton Schools of Engineering at Arizona State University…