ASU research finds better predictors of metal structure failures
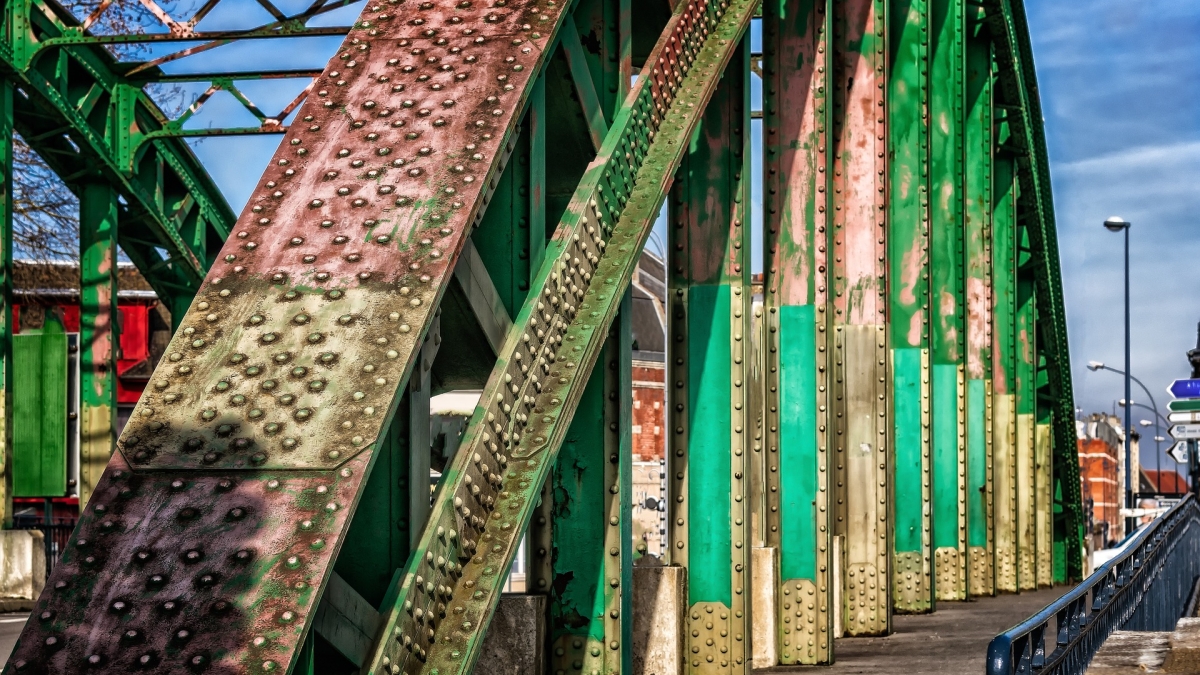
Stress-corrosion cracking is an environmental cause of premature failure in engineered structures, including bridges, aircraft and nuclear power generating plants. Photo by Pixabay
An Arizona State University research team has released new insights about intergranular stress-corrosion cracking (SCC), an environmental cause of premature failure in engineered structures, including bridges, aircraft and nuclear power generating plants.
The research, “Decoupling the role of stress and corrosion in the intergranular cracking of noble alloys,” released Monday in Nature Materials, addresses the assumption that intergranular SCC is the result of the simultaneous presence of a tensile stress and corrosion and demonstrates that the roles of stress and corrosion can be decoupled, or can act independently.
“The finding is the culmination of about 30 years’ work on this kind of stress corrosion problem,” said lead researcher Karl Sieradzki, a professor of materials science and engineering at ASU. “We now have a view into how new alloys can be designed to avoid this form of stress corrosion-induced failure.”
When metals are exposed to water containing salts, the strength of the metal can be severely compromised and lead to unexpected failure. An example of an SCC failure is the 2003 Kinder Morgan gasoline pipeline in Tucson, Arizona.
The conventional paradigm for understanding SCC conditions is the simultaneous presence of a sufficient level of tensile stress, a corrosive environment and a susceptible material.
The research challenges this viewpoint and illustrates that the simultaneous presence of stress and a corrosive environment is not a requirement for SCC, and that it can occur if the corrosion happens first and the material is subsequently subjected to stress.
Karl Sieradzki
In addition to Sieradzki, the paper’s authors include Nilesh Badwe, Xiying Chen, Erin Karasz and Ariana Tse from ASU and Daniel Schreiber, Matthew Olszta, Nicole Overman and Stephen Bruemmer from Pacific Northwest National Laboratory. The research was supported by the U.S. Department of Energy.
The team examined the behavior of a laboratory model silver-gold alloy, which mimics the corrosion behavior of important engineering alloys, such as stainless steels and nickel-base alloys used in nuclear power plants.
Corrosion in these engineering alloys, as in the model silver-gold alloy, results in the formation of nanometer-sized holes within the corroded layer. According to Sieradzki, the key parameter determining the occurrence of rapid SCC is the adhesion between the corroded layer and the un-corroded alloy. Using the atomic-scale techniques of high-resolution electron microscopy and atom probe tomography, together with statistical characterizations, the team determined that the apparent requirement for the simultaneous presence of stress and corrosion exists because of time-dependent morphology changes that affect adhesion.
As long as adequate adhesion between the layers is maintained, a crack that starts with the corroded layer may penetrate into the uncorroded alloy. This means that there can be a significant mechanical component to stress corrosion cracking that cannot be identified by any measurement of corrosion. The result is that a corrosion measurement can underestimate the rate of SCC by multiplicative factors of 10 or more.
“In nuclear plants, SCC maintenance and plant shut downs are based on previous experience with similarly designed reactors,” Sieradzki explained. “While we are not building new nuclear plants in the U.S., these findings should trigger the search for new, corrosion resistant alloys that can be used for replacement parts in existing plants and in other important structural applications.”
More Science and technology

ASU water polo player defends the goal — and our data
Marie Rudasics is the last line of defense.Six players advance across the pool with a single objective in mind: making sure that…

Diagnosing data corruption
You are in your doctor’s office for your annual physical and you notice the change. This year, your doctor no longer has your…
Large-scale study reveals true impact of ASU VR lab on science education
Students at Arizona State University love the Dreamscape Learn virtual reality biology experiences, and the intense engagement it…