ASU program partners with scouts in Nicaragua on drug and conflict intervention program
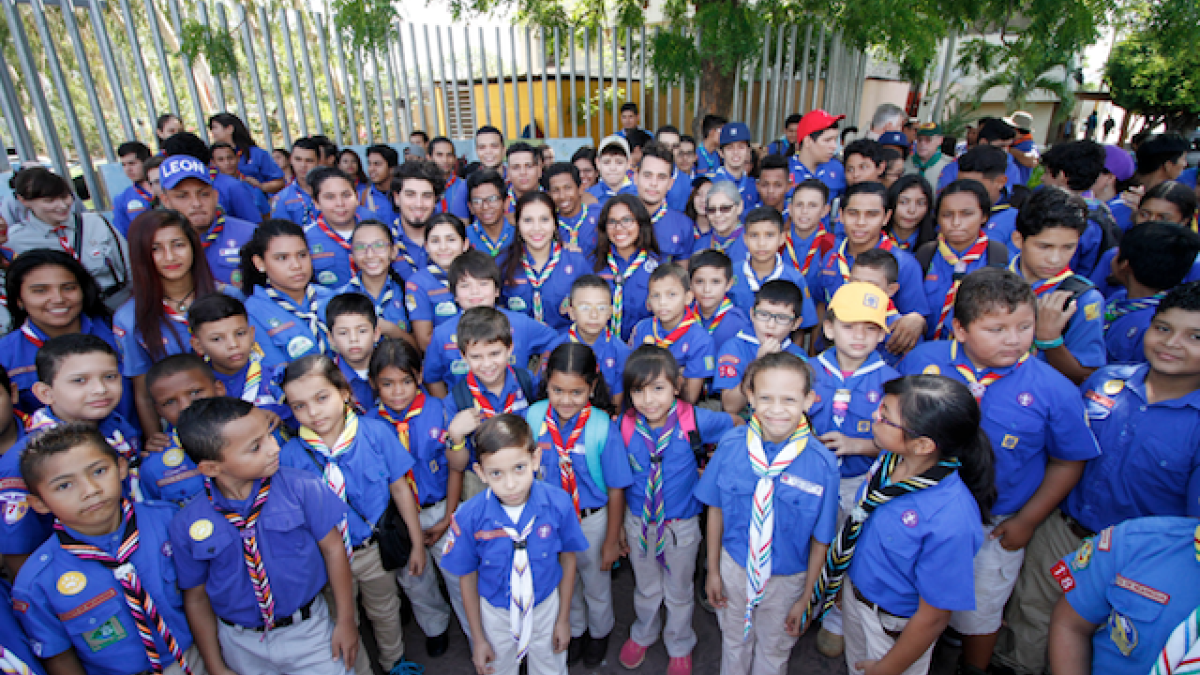
Asociación de Scouts de Nicaragua. Photo by Televicentro (Canal 2)
In recognition of the need to curb drug use and violence for adolescents, the president of the National Scouting Association of Nicaragua signed a partnership agreement with Dale se REAL, an Arizona State University program that teaches young people practical ways to handle situations involving drugs and conflict.
The project is led by Hugh Downs School of Human Communication Assistant Professor Jonathan Pettigrew. It has received funding since 2013 from the Bureau of International Narcotics and Law Enforcement Affairs, a division of the U.S. State Department.
Dale se REAL is a program customized by Nicaraguan youth, for Nicaraguan youth. The program builds on the best drug and violence prevention practice in the U.S. and has adapted it to fit the Nicaraguan context.
The story of the partnership agreement between the scouting program and ASU was recently covered on a national television station in Nicaragua.
“It was the scouts who called the news agency to witness the signing, which indicates the value they see in Dale se REAL and its role in their mission to support youth development,” Pettigrew said.
Dale se REAL teaches middle school students in 47 schools and organizations in Nicaragua skills for developing healthy relationships. The program also surveys some of the students in these institutions to learn about their family experiences, parent-child communication, and health behaviors like drinking alcohol or bullying. This enables Pettigrew and his team to examine direct and indirect associations between family bonding and adolescent alcohol use.
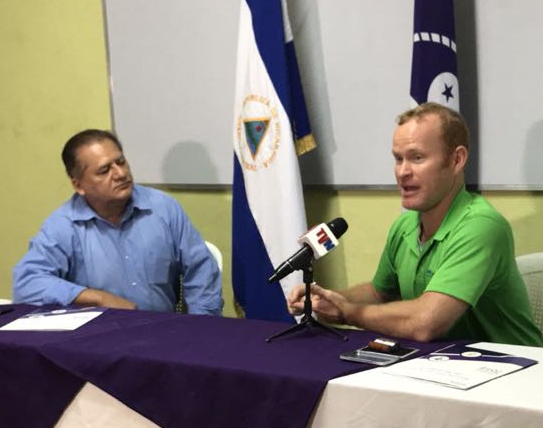
Jorge Katín, president of the Asociación de Scouts de Nicaragua at a news conference with Josh Allsup, national director of Dale se REAL.
“We have discovered that it is the quality of direct communication about drugs and alcohol between parents and children that predicts adolescents' anti-drug perceptions, rather than the quantity," said YoungJu Shin, assistant professor in the Hugh Downs School who served as co-investigator for the grant project. "One good conversation between a parent and a child can be better than a series of conversations.”
“Nicaraguans face unique substance-related challenges,” Pettigrew added. “For one thing, there is no legal drinking age, per se. The legal age for purchasing alcohol there is 18, but it is rarely enforced. Alcohol is relatively inexpensive and readily available. It is the most commonly used substance, which has led to numerous alcohol-related deaths. Because of this, the role that parents and prevention programs like Dale se REAL play in deterring teenage drinking is extremely valuable.”
Pettigrew is optimistic about the expansion of the program through the scouting program in Nicaragua. “Because of this agreement, hundreds more Nicaraguan youth will develop skills to keep them healthy throughout their lives.”
More Local, national and global affairs
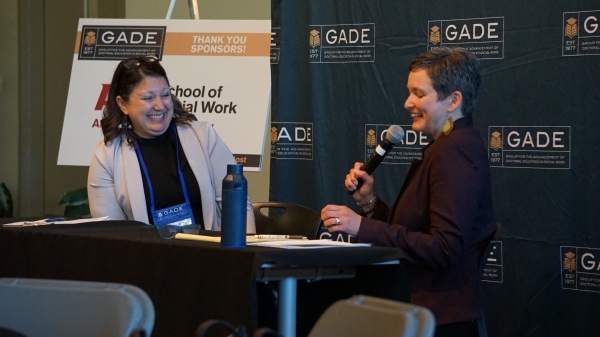
Sustainability takes spotlight at ASU-hosted social work educators conference
The academic discipline of social work not only educates future social workers who help people cope with life’s challenges — it…
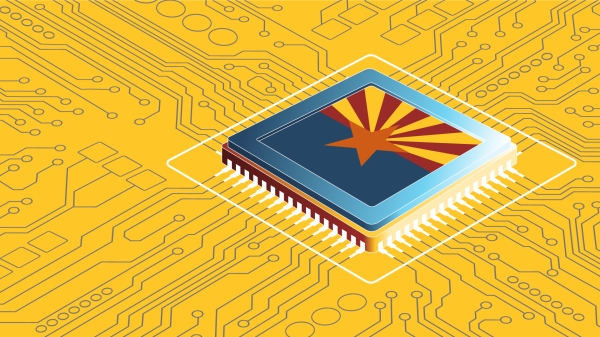
ASU at the heart of the state's revitalized microelectronics industry
Gradually, and then suddenly. It’s not only a famous line from Ernest Hemingway’s novel “The Sun Also Rises,” it’s an apt…
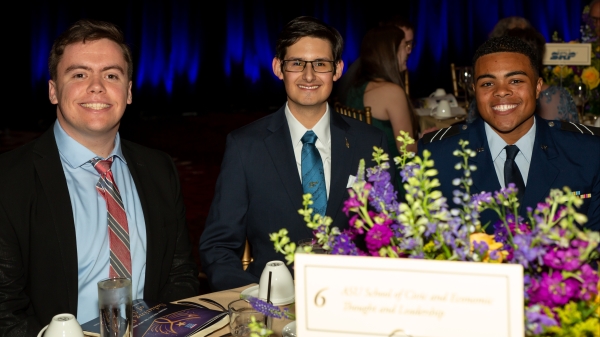
ASU class connects students with veterans
A brand-new class offered this spring through Arizona State University's School of Civic and Economic Thought and Leadership is…