ASU scientists partner in new enzyme library to study elusive glycan molecules

Glycans play a critical role in health and disease (particularly cancer), though they have been notoriously tricky to study in detail. Graphic by Jason Drees/ASU Biodesign Institute
Glycans are essential to virtually every biological process in the body. These complex structures — composed of interlocking sugar molecules — adorn the surfaces of cells in fuzzy profusion. Glycans are a crucial part of a cell’s identity, helping it communicate with other cells and with the external environment. Glycans are also known to play a vital role in cancer, autoimmune disease and countless other afflictions.
Despite their ubiquity and importance, glycans remain among the most enigmatic biological players.
Now, researchers from Arizona State University’s Biodesign Institute join an international research group to probe the deeper mysteries of glycan structure and function. To do this, they have assembled a library of the enzymes necessary to create, modify and degrade glycans. Using this library, the group was able to express these enzymes in two types of cellular host — mammalian and insect.
“It is clear that these elaborate sugar structures play critical roles in both health and disease,” said Joshua LaBaer, director of the Biodesign Institute and Virginia G. Piper Center for Personalized Diagnostics.
He went on to say that science still has very little understanding of glycan activity, because unlike other processes in biology, they are not assembled based on a template. Instead, they are produced by a complex interplay of a large family of enzymes that add and remove specific sugars depending on several factors.
“For the first time, we have built and assembled cloned copies of all the enzymes in this family, creating an invaluable toolkit that researchers will be able to use to build and test these structures,” Labaer said.
Results of the new study, which appear in the current issue of the journal Nature Chem Bio, have potentially broad impact in areas ranging from new diagnostics and therapeutics for disease, to other advances in health, materials science and energy.
Life is sweet
“Studying glycan structures in animal cell systems has historically been a big challenge, especially in terms of technology,” said Kelley Moremen from the University of Georgia, lead author of the new study. “In order to understand how these molecules are made and regulated in terms of function at the cell surface, it’s important to understand the enzymatic machinery that makes them, modifies them and breaks them down.”
One of four major classes of macromolecules making up living systems, (along with nucleic acids, proteins and lipids), glycans are essential for cell structure and function. They play critical roles in cell signaling, immunity, and inflammation.
For example, glycans on cell surfaces are critical for molecular recognition, guiding white blood cells through the body to sites of infection, enabling the immune system to respond where needed. This dynamic is clearly seen during the early stages of flu infection, where the influenza virus particle attaches to a human host cell by recognizing and binding with cell-surface glycans.
The immune system responds to such threats by learning to recognize the glycan sugar coating of viruses, bacteria and other invaders, mounting both innate and adaptive defense mechanisms.
Various forms of sugar are essential to life. Glucose, one of the most important, is a primary metabolite providing energy to the brain. It also exemplifies the two-edged nature of complex sugars, as its dysregulation is a central risk factor for the development of cardiovascular disease. Diabetes, for example, results from improper glucose control by normal metabolic mechanisms. High concentrations of glucose can cause serious organ damage, while low concentrations can lead to loss of consciousness and sudden death due to inadequate energy.
Elsewhere, the walls of plant cells are primarily composed of glycans and account for the planet’s dominant source of biological carbon sequestration, or biomass. They represent a largely untapped sustainable source of non-fossil fuel-based energy.
Until now however, glycans have not enjoyed the same level of scientific attention as nucleic acids or protein and much about their subtle range of duties remains shrouded in mystery. The reason is that the dizzying assortment of glycan structures has, until recently, been notoriously difficult to study.
Genes to proteins
Much of the machinery of life is well understood, at least in broad outline. Different arrangements of the four nucleic acids in DNA form genes that are first transcribed into RNA, then translated into proteins, according to a strict regimen.
Glycans are different. They are not produced from templates like RNA and protein, but instead, are assembled on the fly as needed according to complex factors in the environment including cellular metabolism, cell type, developmental stage, nutrient availability and many other cues. Specialized cells like nerve, skin or muscle cells have their own unique complement of glycans and diseased cells typically display characteristic abnormalities in the glycans adorning them. To produce this richness of glycan diversity, a large number of specialized enzymes are called into service.
Glycans typically attach to specific locations on proteins, modulating their biological activity through molecular recognition or affecting their circulation time in the bloodstream. Glycosylation — the addition of glycan molecules — is one of the most important regulatory mechanisms affecting proteins after they have already been translated from RNA. Such post-translational modifications allow a fairly modest number of human genes — just 25,000 or so — to generate the astonishing complexity and diversity observed in humans and across human populations.
Virginia G. Piper Center for Personalized Diagnostics
This new insight into proteins as highly dynamic entities has revolutionized modern biology, though it has significantly deepened the complexities researchers face. The tidy model of 1 gene generating 1 RNA sequence yielding a single protein of known structure and function has given way to a world of subtle and dynamic protein modification, having profound implications for human health and disease. The study of aberrantly formed glycans, for example, is now a major new avenue of cancer research.
Designing with sugar
But how are glycans made? The process begins when simple sugars in foods — known as monosaccharides— are consumed. These monosaccharides travel to two key subcellular compartments known as the Golgi complex and the endoplasmic reticulum. These membrane-bound organelles act as factories for the stepwise assembly of monosaccharide building blocks into complex, branching glycan structures.
Glycans are then attached to proteins or lipids and delivered to the plasma membrane, coating cell surfaces with these sugar molecules. It has been estimated that 70 percent of cell surface proteins are glycosylated and researchers are still trying to establish the function of all these glycosylations.
Applications of such research may include glycan-based techniques for early detection of cancer and other diseases, and the development of future vaccines and pharmaceuticals against infectious diseases based on a better understanding of host-pathogen interactions and the immune response. The creation of new products or fuels composed of carbohydrate raw materials may also arise from advances in glycobiology.
Library access
In the current study, researchers successfully produced the full range of glycan-forming and modifying enzymes responsible for producing over 7000 vertebrate glycan structures. To accomplish this, the study attempted simplify the process, producing stripped-down enzyme versions capable of effective expression in host cells.
“With the help of Jason (Steele) and Josh (LaBaer), we created a design strategy to capture these coding regions and put them in a holding vector,” Moremen said. “Then, we were able to slot them into insect or mammalian cells and test their ability to be expressed in those systems.”
This is an important advance. Previous efforts to produce and express glycoenzymes in simpler bacterial cells like E. coli had largely run aground. To manage the feat in more complex eukaryotic cells, like those of insects and mammals, considerable modification of the enzyme coding genes was required before their insertion into specialized vectors known as cassettes. These glycoenzyme-coding cassettes were then inserted into insect and mammalian cells, where they were expressed.
The group produced a comprehensive list of 339 glycoenzymes responsible for formation, modification and degradation of glycans, targeting these for protein expression. While the results showed successful, high-level expression of glycoenzymes in both insect and mammalian cells, the authors note distinct differences in specific expression levels in each of the two model systems.
The resulting comprehensive library of glycoenzymes provides a vital resource for future advances in glycobiology and is available to researchers worldwide through DNASU.
An enhanced appreciation of glycan structure and function, explored with the aid of the new enzyme library, will help to advance a number of exciting domains of research, including genomics, proteomics, chemical synthesis, materials science, and engineering.
More Science and technology

Cracking the code of online computer science clubs
Experts believe that involvement in college clubs and organizations increases student retention and helps learners build valuable…
Consortium for Science, Policy & Outcomes celebrates 25 years
For Arizona State University's Consortium for Science, Policy & Outcomes (CSPO), recognizing the past is just as important as…
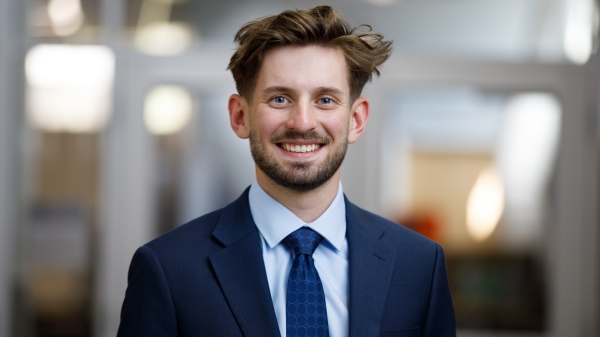
Hacking satellites to fix our oceans and shoot for the stars
By Preesha KumarFrom memory foam mattresses to the camera and GPS navigation on our phones, technology that was developed for…