ASU Polytechnic team wins wildlife Quiz Bowl
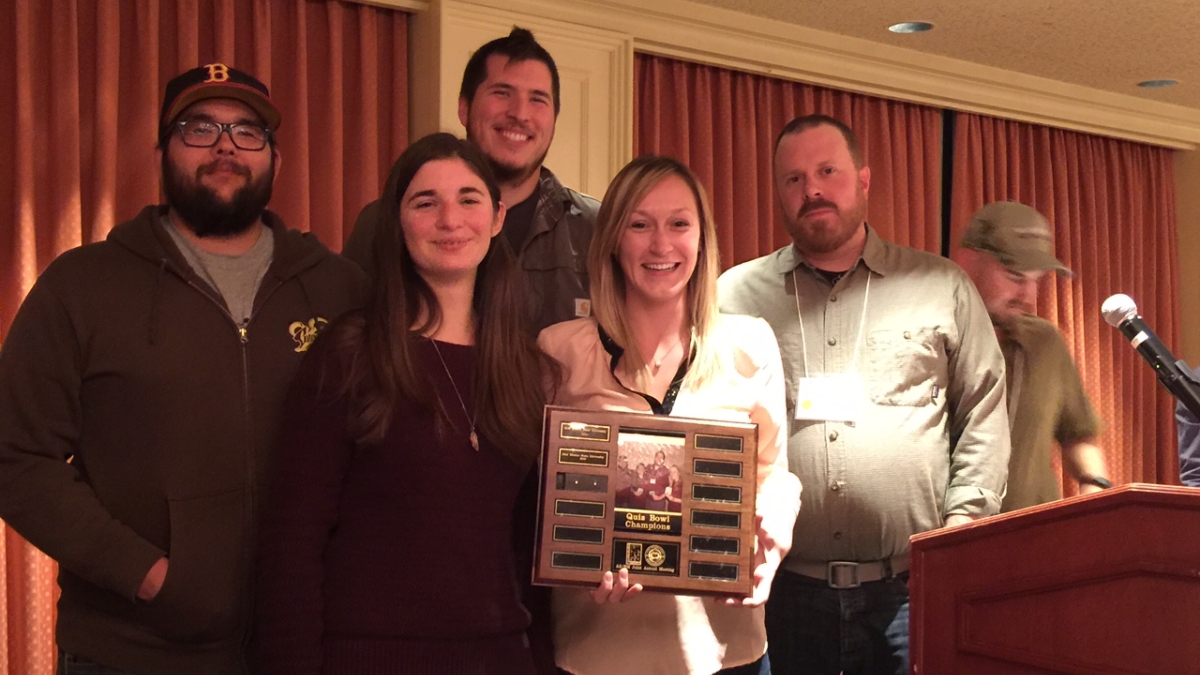
ASU Polytechnic team members (from left) James Ecton, Jacquie Evans, Sky Arnett-Romero and Jessica Latzko accepted the Quiz Bowl "traveling plaque" from Quentin Hays (far right), president of the New Mexico Wildlife Society. The award was presented in front of the 400 professionals and students who gathered for the joint annual meeting of the Arizona – New Mexico Chapters of the American Fisheries Society and The Wildlife Society.
Buoyed by the thrill of participating in the 2013 national wildlife trivia Quiz Bowl, held in conjunction with the annual conference of The Wildlife Society, four ASU Polytechnic natural resource management students returned from that contest with a big idea.
What if a similar Quiz Bowl event could be offered at the regional level?
“Held closer to home, it would give more students the chance to compete — and also be a hook to get students engaged with the professional community of researchers and practitioners in wildlife biology and habitat management,” said Heather Bateman, associate professor of applied biological sciences in the College of Letters and Sciences, who took up the cause and chaired the committee that launched the first regional Quiz Bowl competition in 2014.
Three years later, the contest has become quite popular with student chapters, which now have the chance to compete at the Joint Annual Meeting of the Arizona and New Mexico Chapters of the American Fisheries Society and The Wildlife Society.
Last week, when the societies gathered for their 49th joint annual meeting at the Little America Hotel in Flagstaff, Arizona, from Feb. 4-6, the Quiz Bowl competition drew nine teams: one from Northern Arizona University, one from Prescott College, two from New Mexico State University, two from Eastern New Mexico University, two from Bosque School and one from ASU.
Coming full circle, this year’s Quiz Bowl committee was chaired by a member of that first ASU team to compete at nationals, alumnus Brett Montgomery, now with the Utah Division of Wildlife Resources.
ASU’s 2016 team of James Ecton, Jacquie Evans, Sky Arnett-Romero, and Jessica Latzko — all applied biological sciences majors from ASU’s natural resource ecology program in the College of Letters and Sciences at the Polytechnic campus — finished strong in every round, earning a place in the finals.
“It was a repeat of last year’s final match-up — we once again faced a team from New Mexico State,” said Latzko, president of the Wildlife and Restoration Student Association at the Polytechnic campus and a veteran of the 2015 ASU team which took home second place.
But this year the Sun Devil team prevailed.
“None of us thought we would do as well as we did; we were just hoping that we didn't get crushed,” said teammate Jacquie Evans, who is president of ASU’s student chapter of the Society for Range Management. “We realized that we may have a chance when we watched the first round and knew almost all of the answers between the four of us.
“The win was truly a team effort,” Evans continued. “We each had our own areas of expertise: Sky covered the reptile questions; Jessica got the fisheries questions; I knew about birds and plants; and James provided support, random trivia, and a really important quick reflex.”
The finals drew a standing-room-only audience of more than 150 people — all on tenterhooks — for a contest in which teams not only need to know the right answer but must be quick to buzz in.
“I was on the edge of my seat, trying to hide my emotions and had my hand over my mouth so I wouldn’t accidently ‘mouth’ any answers,” said ASU’s faculty coach, Stan Cunningham, wildlife biology lecturer on the Science and Mathematics Faculty in the College of Letters and Sciences. “It was tight but it was a good win.”
Cunningham, who also chaired one of the wildlife panels at the meeting, said he received numerous compliments on the caliber of ASU’s students, their overall turnout at the meeting (17 students attended), and the ASU Polytechnic program.
“This type of recognition does a lot for our program and, more importantly, our students’ ability to compete for professional positions,” he said. “We have an excellent natural resources ecology curriculum at the Polytechnic campus. Our students have done well with it, and it's nice to see it gaining visibility.”
In addition to competing, students participated in the full range of conference events, including fisheries and wildlife sessions focused on new research, technology, best practices, and the impact of climate change; poster sessions; as well as a timely plenary session, “Who Will Manage the Future of Our Public Lands,” which brought together elected officials, policymakers, conservation activists, and USDA Forest Service practitioners.
“I was glad so many of our students were able to be at these meetings and attend a plenary devoted to a current political and public policy debate that will directly impact their careers and our wildlife,” said Cummingham.
Latzko said she greatly appreciates the friendships forged in competition. "Attending the conference the last three years, I've gotten to know some of the other students from different schools that we competed against. I think that’s what makes it fun! It's a healthy competition among friends — one that I think we'll look back on fondly when we're in our professional careers and likely still coming to the conference."
More Science and technology
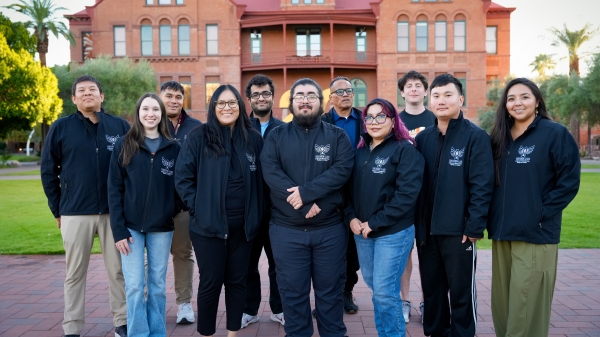
Indigenous geneticists build unprecedented research community at ASU
When Krystal Tsosie (Diné) was an undergraduate at Arizona State University, there were no Indigenous faculty she could look to…

Pioneering professor of cultural evolution pens essays for leading academic journals
When Robert Boyd wrote his 1985 book “Culture and the Evolutionary Process,” cultural evolution was not considered a true…

Lucy's lasting legacy: Donald Johanson reflects on the discovery of a lifetime
Fifty years ago, in the dusty hills of Hadar, Ethiopia, a young paleoanthropologist, Donald Johanson, discovered what would…