X-ray laser resolves atomic structure of biomolecules
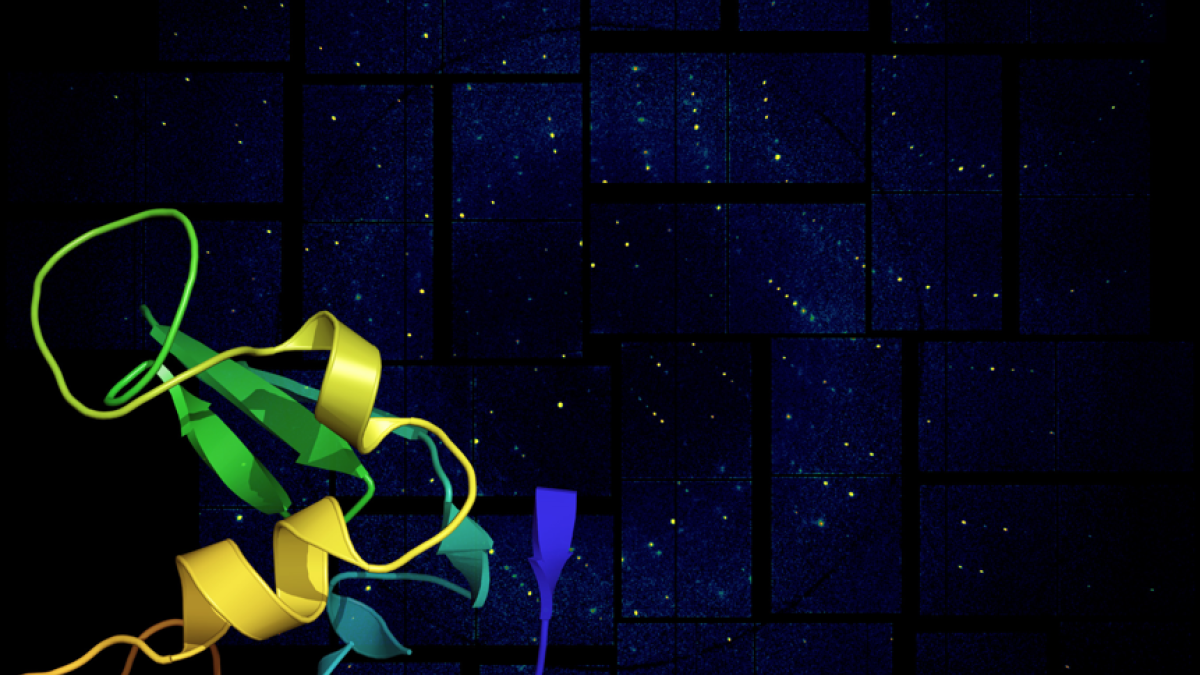
An international team, led by the U.S. Department of Energy's SLAC National Accelerator Laboratory and including members from Arizona State University, has shown how the world's most powerful X-ray laser can assist in cracking the atomic code of biomolecules, including a small protein found in egg whites.
The team’s experiments, described this week in Science, used SLAC’s Linac Coherent Light Source (LCLS) to obtain ultrahigh-resolution views of nano-crystals of biomolecules. In the process, the work is helping pioneer critical new investigative avenues in biology.
“This work demonstrates a new ‘snap-shot’ X-ray laser method that can provide atomic-resolution images of proteins with femtosecond time resolution and without causing radiation damage of the sample,” said John Spence, an ASU Regents' Professor of physics.
"This is the first high-resolution demonstration of the ‘diffraction-before-destruction’ technique on biological samples, where we’re able to measure a sample before the powerful pulses of the LCLS damage it,” added Sébastien Boutet, a staff scientist at LCLS who led the research. “We were able to actually visualize the structure of the molecule at a resolution so high we start to infer the position of individual atoms,” Boutet added.
For decades, scientists have reconstructed the shape of biological molecules and proteins by illuminating crystallized samples with X-rays to study how they scatter the X-rays. The new work with lysozyme (egg white protein) represents the first high-resolution experiments employing serial femtosecond crystallography – the split-second imaging of tiny (submicron) crystals using ultrashort, ultrabright X-ray laser pulses. (A femtosecond is one quadrillionth of a second.)
The technique – described in the paper “High-resolution protein structure determination by serial femtosecond crystallography” – works at a higher resolution than previously achieved using X-ray lasers, allows scientists to use smaller crystals than typical with other methods, and could enable researchers to view molecular dynamics at a time-scale never observed before.
The team chose lysozyme as the first sample for their research because it is easy to crystallize and has been extensively studied. Their work determined lysozyme’s structure at such high resolution that it showed the individual amino acids which make up the protein molecules, of which living organisms mainly consist. At this resolution, the researchers could tell the difference between the almost identical amino acid sequences of a turkey and of a hen lysozyme.
Spence said the team has previously published the structure of much larger and more complex proteins using LCLS but at lower resolution, such as photosystems used in photosynthesis, which were made at ASU in the laboratory of professor Petra Fromme.
International team members in addition to ASU and SLAC includes researchers from Max Planck Institutes, DESY, Cornell University, State University of New York-Oswego, the Applied Physics Laboratory at Johns Hopkins University, the Nikhef National Institute for Subatomic Physics, the European Synchrotron Radiation Facility, University of Gothenburg, University of Hamburg, University of Lübeck and Uppsala University.
In addition to Spence, other ASU members of the team are Bruce Doak, professor of physics; Petra Fromme, professor of chemistry and biochemistry; Uwe Weierstall, research professor in physics; Raimund Fromme, research associate; Richard Kirian, graduate student; Mark Hunter, graduate student; Christopher Kupitz, graduate research assistant; D. Wang, graduate student and Nadia Zatsepin, post-doctoral researcher. The ASU group developed the sample delivery system and did the early development work on the new “Monte Carlo” data analysis method. All ASU participants are members of the College of Liberal Arts & Sciences.
“These results show that 3D image of molecules can now be obtained at atomic resolution by our new X-ray snap-shot method, in which we collect the scattering for the image before the sample is later destroyed by the beam,” Spence said. “By ‘outrunning’ radiation-damage processes in this way, rather than by freezing the sample, we can record the time-evolution of molecular processes at room temperature. This opens the way to future experiments on laser-excited samples, 3-D image reconstruction and a host of other experiments on fast imaging, all directed at the grand challenge of obtaining movies showing molecule machines at work.”