Big insects provide big answers about oxygen
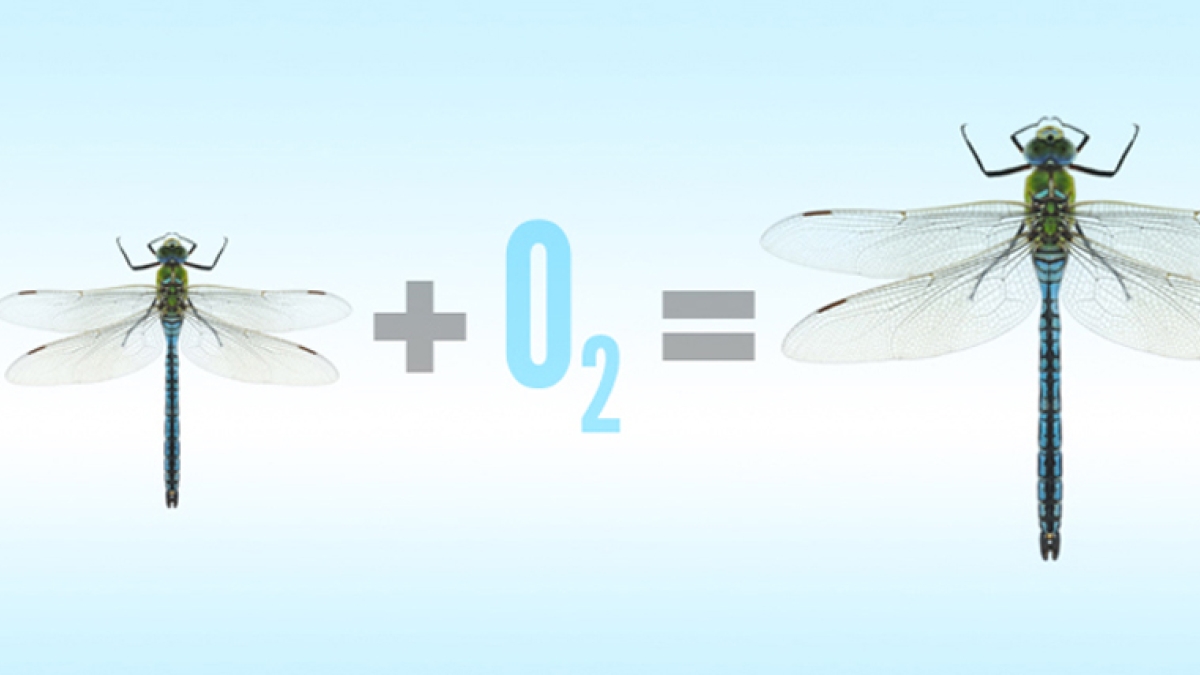
Giant insects are something that would terrify most rational human beings. Fortunately, such things only exist in the realms of science fiction and horror, right? Actually, no.
John VandenBrooks, a post-doctoral research associate in ASU’s School of Life Sciences, has raised dragonflies that were 15 percent larger than their normal size. But why would someone want to bring supersized bugs into the world?
Simply put, VandenBrooks is interested in how an animal’s environment affects its evolution.
Approximately 300 million years ago, creatures on Earth lived in oxygen rich environments. These high oxygen levels peaked around 31 percent, compared to 21 percent oxygen in our atmosphere today. According to the fossil record, this resulted in some pretty big bugs.
These giant insects lived in the Carboniferous period, and included dragonfly-like creatures known as Protodonata, with wingspans almost three feet wide. Beaked insects called Palaeodictyoptera had wingspans of 22 inches, and millipedes stretched more than six feet long!
In order to determine how oxygenation affects animals’ development, VandenBrooks used a three-pronged approach. He studied the fossil record of these behemoth insects, reared modern analogues in high oxygen environments and developed a potential method to determine oxygen levels of the past.
VandenBrooks’ interest in oxygen’s effect on evolution and physiology goes back to when he started his doctorate at Yale University. While working with fossils in paleontological digs and museums, he found himself without a reference point.
“I thought to myself, ‘it’s really hard to interpret any of this, because we don’t have an understanding of how oxygen levels affect anything today. So how are we supposed to understand what’s going on in the fossil record if we don’t understand what’s going on now?’” says VandenBrooks.
To answer this question, VandenBrooks began rearing alligator eggs in varied concentrations of oxygen and analyzing the development of their embryos. The changes he saw in modern alligators correlated with those in the fossil record. Unfortunately, alligators take 17 years to fully mature, making them less than ideal for laboratory work. He needed to use organisms with shorter generational spans.
VandenBrooks began rearing insects in three different environments, imitating various oxygen concentrations throughout time: a 12 percent oxygen atmosphere, Earth’s estimated lowest level; today’s 21 percent; and the oxygen-saturated 31 percent of the Carboniferous period.
The first insects VandenBrooks and other ASU researchers raised were fruit flies and beetles. The latter showed an increase in body size in response to more oxygen.
“We really wanted to apply this to groups that are more applicable to fossil record,” said VandenBrooks. “So that’s when we started on the cockroaches and dragonflies.”
Mirroring their formidably sized Protodonata predecessors, the dragonflies responded to the high oxygen levels in kind, swelling to 15 percent larger than those reared in normal oxygen levels. In low oxygen, meanwhile, the dragonflies ended up 20 percent smaller than today’s typical size.
To the relief of entomophobes everywhere, the cockroaches did not increase in size at all. However, they did respond to the extra oxygen in different ways. Namely, it affected their respiratory system, growth, development and fecundity.
“Even if they’re not showing any changes in body size, there are definite impacts on their physiology,” says VandenBrooks.
The most notable change he observed was that the roaches developed smaller tracheae, hollow tubes that deliver gases to tissues. Because they were in an oxygen rich environment, the insects could develop thinner tracheae to distribute the needed amount of oxygen. This freed up the roaches to invest more in other systems and become more efficient, competitive animals. This ability, in which an organism can change its physical properties or traits to better suit its environment, is known as “phenotypic plasticity.”
“Conversely, if oxygen is low, they invest more in that tracheal system and have less room to invest in those other tissues,” says VandenBrooks.
The tracheae of the cockroaches were measured at Argonne National Laboratory in Illinois, where researchers generated high-powered, high-resolution x-ray images of the insects’ tracheal systems. Through this work, VandenBrooks is developing a method for determining what the widely disputed oxygen levels of the past were.
“There are five competing models for ancient oxygen levels and the only thing that they agree on is that oxygen was high when the dragonflies were big and low after that,” says VandenBrooks. “What the values were, we don’t really know.”
By measuring tracheae, it’s possible to draw a correlation with the concentration of oxygen at a given time. Well-developed tracheae indicate that oxygen levels were low. Thinner tracheae, on the other hand, suggest a high oxygen environment.
This process of measurement, or proxy, is similar to the way scientists estimate historical levels of carbon dioxide in the atmosphere. They examine stomata, openings on plants that allow water, oxygen and carbon dioxide to pass through. Lots of stomata reveal a low carbon dioxide level, while few stomata indicate high levels of the gas. In determining oxygen levels, the roaches’ investment in tracheal systems is a lot like a plant’s production of stomata.
The rub is that many fossils don’t retain their tracheae. They are simply impressions of an organism in the earth. Fortunately, some ancient insects were trapped in amber – fossilized tree resin. The amber preserves their entire bodies.
Uninterested in returning to paleontological fieldwork, VandenBrooks set out to study fossils that have already been collected. Travelling to the Yale Peabody Museum, the Smithsonian, Harvard Museum of Comparative Zoology and the American Museum of Natural History, he gathered data to compare to similar modern insects.
“I’ve been imaging fossils in amber, thinking that’s my best bet for tracheae,” says VandenBrooks. “And lo and behold, they have tracheae. So if you can measure tracheae of insects in the past, theoretically you could back out and figure out what oxygen concentration was in the atmosphere.”
His next step is to evaluate the performance and behavior of the insects in the oxygen rich environments.
“How does an increase in oxygen affect dragonfly flight? How does it affect cockroach competition?” asks VandenBrooks. “We can see an increase in investment of other tissues, but we don’t have a measurement of the impact of that.”
Using high-speed cameras to monitor leg and wing movements and dissections to weigh musculature, he hopes to answer these questions.
“The second half of the question is mechanism – what’s really the mechanism behind oxygen sensing? How does an insect recognize what the oxygen levels are and how does it know to initiate these changes?” VandenBrooks asks.
While the main thrust of the research is focused on the past, understanding how organisms respond to changing habitats is vital information for today.
“Overall understanding of how evolution happens and how environmental factors influence evolution—that’s relevant,” says VandenBrooks. “There are all these environmental changes going on now, with temperature, carbon dioxide, ecosystem changes and sea level changes. How are these going to impact species and the physiology of animals and plants? It’s really unknown.”
VandenBrooks’ research was co-funded by the National Science Foundation’s Directorate for Biological Sciences and Directorate for Geosciences.
Written by Pete Zrioka, Office of Knowledge Enterprise Development