Advances in X-ray crystallography unveil nature’s tiniest secrets
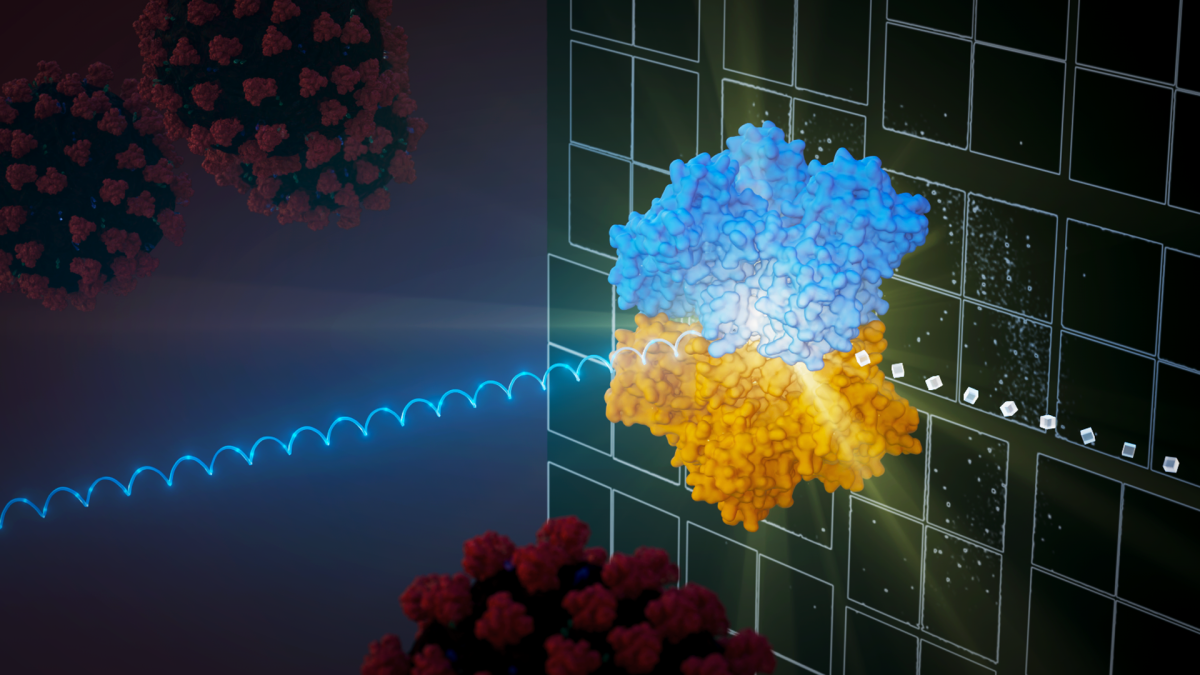
SFX is an innovative method that uses ultra-short X-ray pulses from X-ray free electron lasers (XFELs) to analyze crystallized samples. In this setup, tiny crystals are streamed into the path of the XFEL beam, and as each crystal is hit, a diffraction pattern is produced before the crystal is destroyed. The accumulation of these patterns from numerous crystals allows for the creation of a detailed 3D structure of the biomolecule. Graphic by Jason Drees
A powerful research technique is enabling scientists to peer into the heart of the biological realm with astonishing acuity. Known as serial femtosecond X-ray crystallography, the method is expanding the reach of investigations into how biological molecules interact, yielding insights into the nature of disease and guiding the development of new, smart pharmaceuticals to combat a broad range of ailments.
In a new review paper, Arizona State University lead authors Sabine Botha and Petra Fromme highlight recent advances in the field, focusing on how this approach is deepening the structural understanding of the SARS-CoV-2 virus, the pathogen responsible for the COVID-19 pandemic.
The paper also outlines the unique environment persisting during the COVID-19 emergency, and the challenges of carrying out this highly collaborative and interdisciplinary science while keeping researchers safe.
“This paper is so exciting,” Fromme says. “It enabled us to integrate our review of the recent advances in serial crystallography with XFELs and molecular movies of biomolecules in action. Additionally, it offers insight into how the COVID-19 pandemic altered the approach to conducting highly complex and collaborative experiments at large XFELs.”
Fromme directs the Biodesign Center for Applied Structural Discovery and is the Paul V. Galvin Professor with the School of Molecular Sciences at Arizona State University. Botha is an assistant research professor with ASU’s Department of Physics.
The review appears in a special 30th anniversary issue of the journal Structure.
Petra Fromme
Crystallography at femtosecond speed
Structural biology delves into the intricate designs and forms of biological molecules like proteins and nucleic acids, shedding light on their roles and interactions. This knowledge is essential for crafting effective drugs and treatments for various diseases.
Serial femtosecond crystallography (SFX) has been at the forefront of structural biology over the past decade, pushing the boundaries of what is possible in the study of macromolecules. SFX is special because it allows researchers to take detailed pictures of proteins using very small crystals without damaging them, and it does this at normal room temperatures.
To accomplish this, a stream of tiny, crystalized protein molecules is passed through an extremely bright X-ray laser beam. To give a sense of the speed of the X-ray pulses, millions of them can strike the sample stream in the split second it takes to blink.
Before these proteins are destroyed by the laser's intensity, the method captures their patterns. Researchers gather thousands of these patterns and use computers to reassemble them, producing detailed 3D pictures of molecular structure.
X-ray insights
SFX has deepened the understanding of the delicate architecture of biomolecules that were previously challenging or impossible to study, enabling time-resolved investigations of biological processes with unprecedented precision.
In crystallography, "time-resolved" studies allow scientists to observe the dynamic processes and structural changes that occur in a crystal or biomolecule over extremely short time intervals. Time-resolved crystallography is particularly valuable for investigating fast chemical reactions, enzymatic reactions or conformational changes in macromolecules at the atomic scale.
The SFX method uses special lasers, called XFELs, to gather detailed data from very tiny crystals. The idea of capturing this data before the crystal gets destroyed by the laser was first demonstrated in 2006, leading to the development of SFX. This new method allows scientists to explore biological structures they couldn't before, because the strong X-rays used would damage them.
SFX has been instrumental in studying a wide range of biomolecules, including membrane proteins and fragile protein structures that spontaneously grow inside living cells. It has also played a crucial role in time-resolved studies of enzymatic reactions with unprecedented temporal resolution.
In 2011, an international team of scientists, including Fromme, solved the first protein structure using SFX, revealing the structure of the radiation-sensitive Photosystem I membrane protein complex, a key component in the process of photosynthesis. Further milestones include solving structures of challenging systems like G protein-coupled receptors and membrane proteins as well as enabling time-resolved SFX studies of a broad range of biomolecules.
One factor limiting wider adoption of this powerful tool is the high cost of building and maintaining such instruments, which together runs in the billions of dollars. Only a handful of XFELs exist in the world, and beam time for experiments is limited and fiercely competitive.
That picture may be changing due to a new breed of compact instruments beginning to come online. These include the compact X-ray light source (CXLS) and the compact XFEL (CXFEL) at ASU, which promise to greatly expand accessibility, potentially providing academic laboratories around the world with their own femtosecond source.
Enter COVID-19
The early adoption of SFX advanced with the Linac Coherent Light Source (LCLS) in the United States and other XFEL facilities around the world coming online. These facilities allowed for the expansion of data collection rates into the megahertz range, pushing the boundaries of what could be achieved with SFX. New beamline instrumentation, X-ray detectors and software advancements were developed to support the growing demand for SFX experiments.
However, the rapid progress in SFX faced a significant challenge when the COVID-19 pandemic struck in 2020. The pandemic disrupted scientific research worldwide, including experiments at XFEL facilities. SFX experiments were severely impacted by social distancing and occupancy restrictions.
Before the pandemic, SFX experiments often required the physical presence of large groups working together in subterranean "hutches," making it impossible to conduct experiments safely in a pandemic environment. Travel restrictions, quarantine requirements and limited access to facilities created a daunting situation for scientists.
“When we were invited to write a review on SFX, it was evident to me that we should include the impact of the recent pandemic,” Botha says. “While it is evident that the majority of structural biologists started targeting SARS-CoV-2 proteins to help combat the spread of this resilient virus, the virus positively changed the way we do SFX experiments today. Writing this review was very different from writing a scientific manuscript, because the global pandemic affected all of us on a personal level.”
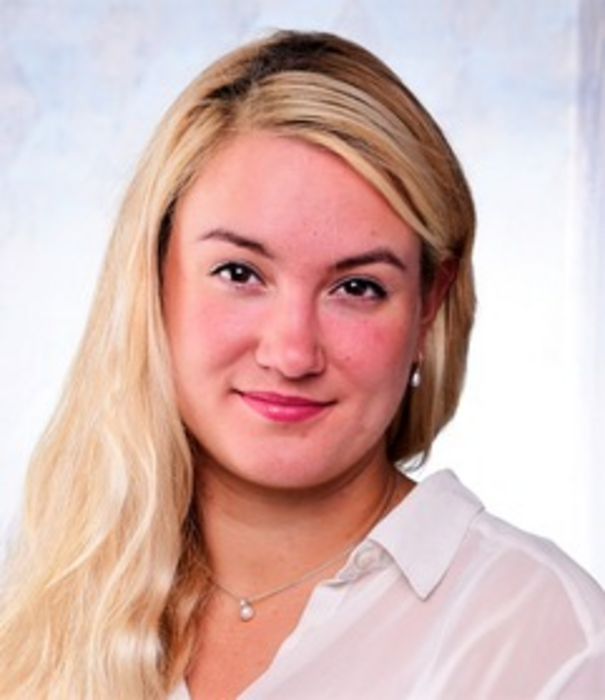
Sabine Botha
In response to the pandemic, XFEL facilities had to adapt quickly to ensure that experiments could continue. Remote operation and data collection, which had been uncommon at XFELs, became essential. The emphasis shifted from having a large team on-site to guiding a select few scientists who were present at the facilities to perform experiments remotely.
XFEL facilities implemented various measures to support remote access, including video conferencing, remote sample injection and data analysis tools. These changes not only allowed ongoing experiments to continue but also significantly reduced the carbon footprint associated with travel.
SFX peers deep into SARS-CoV-2
The pandemic also prompted a shift in research priorities, with many scientists focusing on studying SARS-CoV-2, the virus responsible for COVID-19. XFEL facilities played a crucial role in these efforts by offering rapid-access beam time for SARS-CoV-2-related research.
Experiments at XFELs were conducted to study the structures of key SARS-CoV-2 proteins and potential drug targets. Despite the challenges posed by the pandemic, scientists successfully solved the structures of these proteins, providing valuable insights for drug development.
In one such study, Fromme’s team investigated NendoU, a viral protein used by SARS-CoV-2 to stealthily cover its tracks and shield the pathogen from being targeted by the human immune system.
A new era in structural biology
The adaptability of XFEL facilities during the COVID-19 pandemic has expanded the accessibility of SFX experiments to a broader community of scientists, democratizing the field. Rapidly evolving techniques in the SFX domain promise varied applications, from smart drug design to next-generation clinical imaging and even to areas further afield, including materials science and exploration geology.
More Science and technology
Extreme HGTV: Students to learn how to design habitats for living, working in space
Architecture students at Arizona State University already learn how to design spaces for many kinds of environments, and now they…
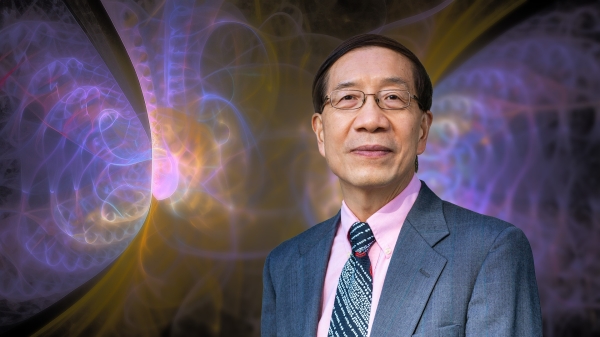
Human brains teach AI new skills
Artificial intelligence, or AI, is rapidly advancing, but it hasn’t yet outpaced human intelligence. Our brains’ capacity for…

Doctoral students cruise into roles as computer engineering innovators
Raha Moraffah is grateful for her experiences as a doctoral student in the School of Computing and Augmented Intelligence, part…