Book explores promise, pitfalls of personalized medicine
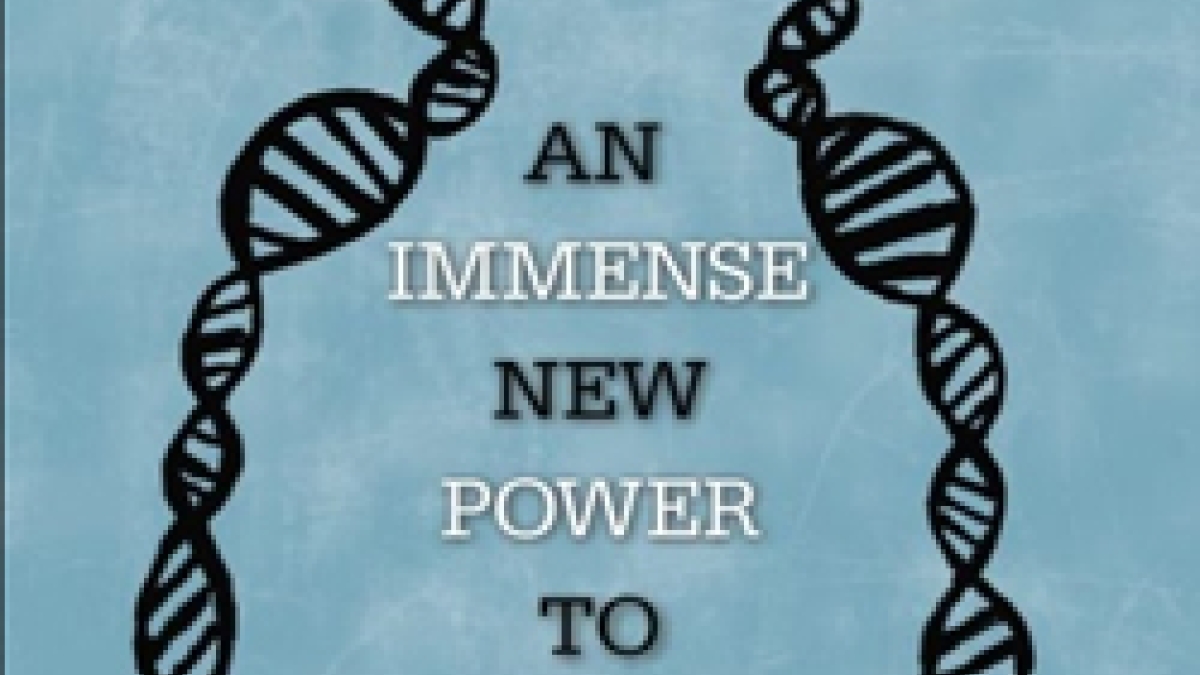
Personalized medicine has promised to radically change the way we look at health and disease. Talk of tailored drug therapies and early detection of cancer has captured the attention of scientists and lay people alike. So when will patients start to reap the benefits of this medical revolution?
The transition to personalized medicine won’t be seamless or swift, says Lee Gutkind, who co-wrote "An Immense New Power to Heal: The Promise of Personalized Medicine" (In Fact Books, May 2012) with novelist and science writer Pagan Kennedy. The authors explain the complex world of personalized medicine in an engaging, approachable storytelling style.
Gutkind is the distinguished writer in residence at ASU’s Consortium for Science, Policy & Outcomes (CSPO) and a professor in the Hugh Downs School of Human Communication. He also is the founder and editor of the journal Creative Nonfiction.
In researching the book, Gutkind learned the tremendous potential for personalized medicine, and the obstacles keeping doctors from putting it into practice.
Giving up turf
One aspect of personalized medicine involves the use of genetics to get a detailed and accurate picture of human DNA and how it responds to disease. When the first complete human genome was sequenced in 2007, scientists gained insight into how genetic variations can predict certain diseases and disorders. The idea is that having access to genetic information will allow health care providers to take a more preventative approach to caring for patients.
However, physicians are traditionally reluctant to adopt new technologies. Most primary care doctors have little training in genetics, and many have never even heard the term “personalized medicine.”
“When I started this book between 2007 and 2008, I could go to physicians in major medical centers across the United States and say the phrase ‘personalized medicine,’ and at least half of those people wouldn’t even know what it was,” Gutkind says. “Again and again, I had to define personalized medicine for the people who ought to have known 10 years ago what it meant.”
Of the physicians who were aware of personalized medicine, few seemed eager to share their knowledge with colleagues and patients. Even most medical schools have yet to amend the curriculum to include genetics and personalized medicine. Gutkind attributes this to a lack of understanding of the importance and potential of personalized medicine. It also speaks to the physician’s unwillingness to “give up turf” to the field of genetics, an area they may know little about.
“We want a revolution in health care, but we are not making the transition,” Gutkind says.
The meaning of genes
It’s understandable that doctors with no training in genetics would be wary of personalized medicine. But Gutkind was surprised to learn that even for a geneticist, garnering useful health information from genetic data is no easy task.
“I really thought we would be able to find a gene or two genes and connect them to all sorts of different diseases, and it would be in fact a simple matter to slowly but surely deal with the biggest challenges in the medical world,” Gutkind says. The reality, he found, is much more complicated.
Imagine being handed a thick book of sheet music and having to comb each line for mistakes. Even if you were a skilled musician, the job would be daunting. That’s similar to what a geneticist must do when examining a sequenced genome. When one does find a genetic variation, it’s rarely obvious what disease or disorder may come of it.
Further complicating the process, a person’s environment and behavior can actually alter their genome. This makes it difficult to determine if a genetic variation is really to blame for a certain disease, or if it is the result of external factors. Additionally, many diseases, including diabetes and most cancers, are caused by small variations in multiple genes, which can be hard to decipher.
Finally, genome sequencing runs about $10,000, which doesn’t include the cost of storing and interpreting the data. While that price is expected to drop significantly in the next few years, Gutkind says there’s no guarantee that our ability to translate genetic data will improve as sequencing becomes more affordable for the average patient.
Scientists have made some progress in linking genetic variations to diseases such as Parkinson’s and Type 2 diabetes. But these connections are not conclusive, and account for only a small fraction of diseases thought to have a genetic link.
Demanding change
Outside of genetics, other aspects of personalized medicine have been applied successfully. Joshua LaBaer, director of the Center for Personalized Diagnostics at ASU’s Biodesign Institute, has been using protein molecules called biomarkers to develop better therapies for breast cancer. Another promising development in personalized medicine is the field of biomedical informatics, which would fully digitize information about patients, medications and research. That way, doctors have easy access to a bounty of information to create more effective and efficient treatment plans.
Genome sequencing may not yet be the game-changer scientists predict. But as research and technology advances, personalized medicine is expected to transform the health care industry and drastically improve our ability to fight and prevent disease. As policymakers continue to debate over health care reform, it’s clear that the system needs revamping.
“We are floundering around, trying to redesign a totally out-of-control health care system, one that inherently has many good things, but is disorganized, unfair and unbalanced in a lot of different directions,” Gutkind says. “Personalized medicine is a very mature and orderly way to reconceive of the system of the world of medicine.”
The question is, how quickly will the transition to a personalized medicine-based health care system take place? Since current and emerging doctors are in no hurry to stray from traditional medical practice, it may be up to patients to demand change.
“We have shown in the past that scientists, government officials and physicians generally are reluctant to make changes. But when citizens – real people – begin to demand and request the change, the government officials and the physicians and the scientists kind of move in that direction,” Gutkind says, citing the AIDS crisis as an example. “Much more research was poured into finding some sort of a cure or controlling agent for AIDS once citizens became advocates for this problem.”
Gutkind hopes that as people read his book and become more informed of the potential for personalized medicine, doctors will be forced to evolve and implement new technology.
One problem is that up until now, most information available on personalized medicine was confusing, boring and not obviously relevant to the average reader’s life. That’s because scientists often have a difficult time explaining their work to a lay audience.
“Scientists are so focused in on what happens to them in the laboratory, they need to be able to connect what they’re doing in the test tube to what’s going to happen when that medication or whatever reaches the bedside,” Gutkind says. “The challenge is to get scientists to think about connecting to a larger audience, and that’s really quite a challenge.”
This is also true of policymakers, whose work can have far-reaching implications but is often obscure and theoretical. That’s why Gutkind leads a workshop called “To Think, To Write, To Publish,” connecting next-generation communicators with policymakers to collaborate in explaining science policy to the public. The program is run by Gutkind and David Guston, director of ASU’s Center for Nanotechnology and Society and co-director of CSPO. It is funded by a $250,000 grant from the National Science Foundation.
“We can make an impact and have an effect when we can take the people who know a lot of stuff, like scientists, and get them to make connections to real people,” Gutkind says.
An Immense New Power to Heal was produced with support from the Virginia G. Piper Charitable Trust. You can buy the book online at amazon.com: http://amzn.to/KYfM4p
Written by Allie Nicodemo, Office of Knowledge Enterprise Development. This article first appeared on Research Matters.