ASU team uses synthetic biology to elucidate the complexities of cell function
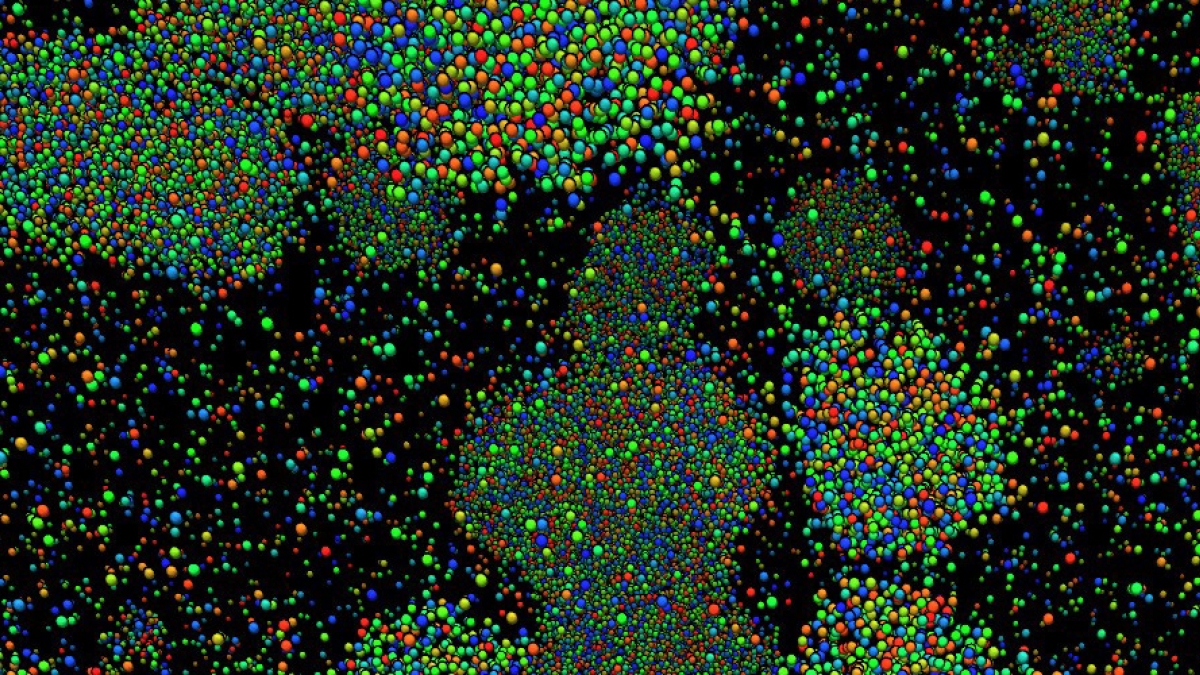
Multi-scale simulations of large-scale systems are used to identify ideal conditions for the formation of membraneless organelles in solution due to the preferred association of functionalized proteins. Computational models range from particle simulations of hundreds of thousands of interacting proteins down to simulations of individual proteins in full atomistic detail.
Arizona State University’s Professor Giovanna Ghirlanda and Assistant Professor Matthias Heyden, both of the School of Molecular Sciences, and Associate Professor Sara Vaiana of the Department of Physics are viewing our cells through a new lens, enabling them to gain novel insight into the mechanisms of several cellular activities.
In addition to membrane-encased organelles — the nucleus, mitochondria and Golgi apparatus, to name a few — eukaryotic cells harbor a variety of compartments that lack a casing. These protein-based liquid globules, called membraneless organelles, carry out various cellular functions that would be less efficient or not possible at all in the cytoplasm. And researchers are now learning that membraneless organelles could play a role in the aggregation of proteins associated with disease, for example Alzheimer’s.
Ghirlanda, Heyden and Vaiana are exploring the biophysics underpinning the formation of these organelles and using the information to design artificial ones. Their research explores an innovative method to achieve compartmentalization, with foreseeable applications in synthetic biology as well as in catalysis.
In the past decade, researchers have learned that a well-known phenomenon known as liquid-liquid phase separation, or LLPS, governs the formation and function of several of these large membraneless structures. The emerging picture of the inside of the cell is that the cytoplasm and nucleoplasm are complex fluids that can stably segregate, similar to oil and vinegar. Borrowing knowledge from fields such as physical chemistry and soft matter physics — where theories explaining liquid-liquid phase separation have informed the development of products ranging from stabilizers in processed foods to cosmetics and from therapeutic ointments to paints — biologists are now developing a new understanding of the nearly two dozen types of membraneless structures characterized so far.
The importance of these structures is becoming increasingly clear. These membraneless structures form through liquid-liquid phase separation of biopolymers such as proteins and RNA, typically in response to a stimulus.
The team is investigating whether LLPS can be exploited to generate designed membraneless organelles capable of performing complex catalytic functions. The applications of these new structures range from improving the efficiency of enzyme cascades in vitro for industrial applications to providing a technically simple, attractive alternative to protein capsules and liposomes in artificial cells.
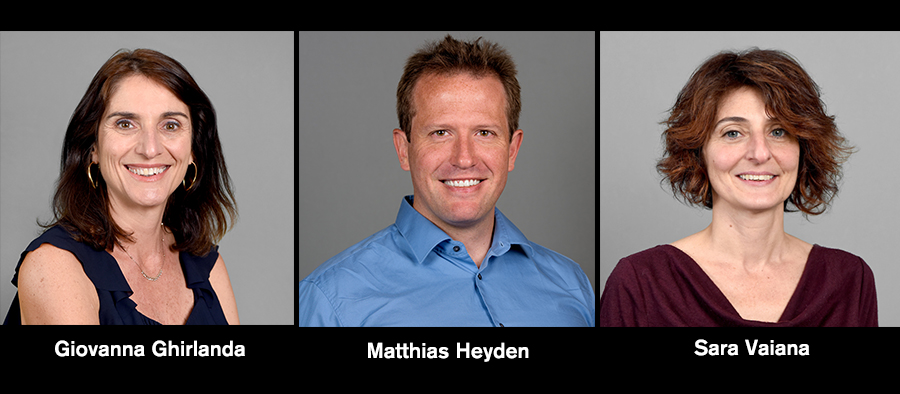
“If successful, our method will result in a straightforward way to obtain membraneless organelles by simply fusing designed tags to chosen enzymes,” said Ghirlanda. “Further, our computational methods will provide a way to tailor the sequences of the tags to the enzymes.”
Concurrent work in the Ghirlanda lab focuses on the design of artificial metalloproteins. In the long term, they aim to generate organelles containing artificial metalloenzymes able to catalyze the production of hydrogen as well as the reduction of carbon dioxide.
Work in the Heyden lab focuses on establishing methods for computer simulation of crowded environments, such as those found in membraneless organelles; the team’s current research has proven a great testing ground for these methods.
The Vaiana lab works on phase separation as it pertains to neurodegenerative diseases. In this context they are establishing biophysical methods to characterize LLPS in vitro and in vivo. They are applying their methods to LLPS to help characterize membraneless organelles, and identify critical interactions underpinning phase transitions for each sequence.
Understanding the mechanism and quantifying the driving forces which lead to amyloid protein aggregation and disease could lead to a desperately needed cure for Alzheimer’s disease.
The trio were recently awarded a National Science Foundation EAGER grant to continue their research. NSF’s EAGER was designed specifically for potentially transformative research that explores new subjects, different methods, or interdisciplinary approaches, exactly what one would expect in innovative research.
In summary, seeing the internal cellular environment as a fluid that contains multiple liquid droplets functioning as membraneless organelles marks a turning point in the understanding of cell biology. The concept is young, and how and why liquid-liquid phase separation organizes the intercellular space remain open questions. But already, it is clear that the phenomenon underpins the formation and functionality of a growing number of long-observed membraneless organelles. With this research, the team aims at exploiting this phenomenon for applications in catalysis and synthetic biology.
More Science and technology
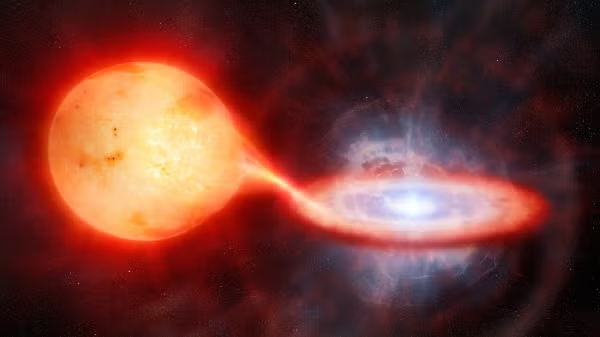
Astronomers observe ultra-hot nova with unexpected chemistry
A team of astronomers, including Arizona State University Regents Professor Sumner Starrfield, has uncovered an exceptionally hot…
Statewide initiative to speed transfer of ASU lab research to marketplace
A new initiative will help speed the time it takes for groundbreaking biomedical research at Arizona’s three public universities…
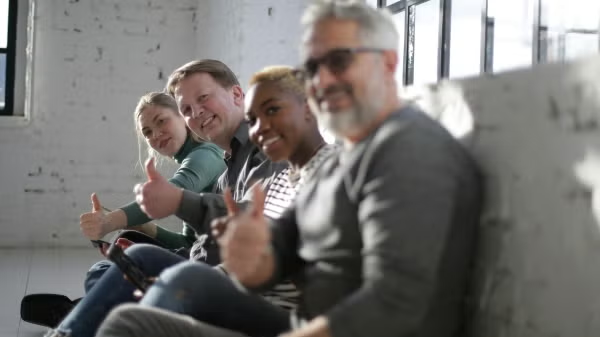
ASU research seeks solutions to challenges faced by middle-aged adults
Adults in midlife comprise a large percentage of the country’s population — 24 percent of Arizonans are between 45 and 65 years…