Useful in a pinch: Nanoscale tweezers are triggered by light

A three-second pulse of ultraviolet light activates tiny tweezers only a few billionths of a meter in size. The light acts to free a trigger strand from its photocage (blue dots) causing the tweezers to spring open. Above are images of the actual nanotweezers in closed and open position, made using atomic force microscopy. Image courtesy of the Stephanopoulos lab and Shireen Dooling
Using segments of DNA, researchers at ASU have constructed a pair of tweezers, measuring 100,000 times tinier than the width of a human hair. A brief burst of ultraviolet light causes the jaws of the tweezers to switch from their closed to open position in seconds.
The new light-activated tweezers are the latest innovation in the fast-moving world of nanotechnology. Devices of this kind, which can directly interact with and influence biological systems, are part of a new breed of nanogadgets that may ultimately be applied in drug delivery, disease treatment, tissue repair and to advance basic understanding of living systems.
The research, which appears in the journal Angewandte Chemie International Edition, was carried out by Nick Stephanopoulos and his colleagues at the Biodesign Center for Molecular Design and Biomimetics at Arizona State University.
Stephanopoulos is a 2018 recipient of the National Science Foundation CAREER Award, the NSF’s most prestigious and competitive honor for early-career investigators.
CAREER awards support early-career faculty who have the potential to serve as academic role models in research and education and to lead advances in the mission of their department or organization. According to the NSF, “activities pursued by early-career faculty should build a firm foundation for a lifetime of leadership in integrating education and research.”
The new award will further support Stephanopoulos’ overarching research goals. The five-year, $548,000 project for the NSF (for which Stephanopoulos will act as principal investigator) is titled “Hybrid protein-DNA nanostructures and devices.”
The laser-activated tweezers described in the new study are just one example of programmable nanostructures capable of interfacing with biological systems, including living cells.
“Biological molecules such as DNA and proteins are the perfect building blocks for making nanoscale devices because they can spontaneously self-assemble into complex structures. Our job as nanotechnologists is to figure out the design rules and synthetic approaches, for making molecules that can form all sorts of interesting shapes and assemblies,” said Stephanopoulos, who is also an assistant professor in ASU's School of Molecular Scienes.
“We are like architects that select from a set of building blocks, figure out the right molecular ‘glue’ we can use to build what we want, and then analyze the properties of the things we make. Cells are full of these fascinating biomolecular nanomachines, so we are constantly ‘borrowing’ both the design principles and the molecules themselves from existing biological systems.”
Stephanopoulos works in the Biodesign Center for Molecular Design and Biomimetics directed by Hao Yan. To date, the center has successfully produced a dizzying array of two- and three-dimensional objects including sophisticated logic gates, nanorobots capable of dynamic activity and nanoarchitectures ranging from Mobius strips to silica-based structures resembling aquatic diatoms.
The new NSF project led by Stephanopoulos has three primary goals: to develop nanofibers (similar to those in tissue) composed of DNA elements linked by proteins, to design and construct three-dimensional cages mimicking viruses from both protein building blocks and DNA scaffolds, and to engineer mechanical elements like hinges or boxes with latches that are activated by proteins.
Augmenting DNA structures with protein components improves their stability and permits interactions with cells that could not be achieved with DNA alone. The proteins could also be used as actuation elements to switch the nanostructures using stimuli such as light. The resultant forms may find their way into gels to stimulate cellular repair, remotely triggered cages for releasing therapeutics to diseased cells and nanorobotic machines for a broad range of applications.
Tiniest tweezers
Scientists would like to design smart, programmable structures capable of interacting with cells and tissues. Such advances will have broad applications in disease diagnostics and treatment, engineering of new tissues and the uncovering of underlying scientific mechanisms.
Researchers coax segments of DNA to form elaborate structures at an incredibly minute scale. The technique, known as DNA origami, relies on the fact that DNA’s four nucleotides, labeled A, T, C and G, stick together in a predictable manner: A nucleotides always pair with T and C nucleotides always pair with G.
Nanoscale architects like Stephanopoulos would like to design DNA-based devices for a range of applications that can function in a stimulus-response manner. Such structures could be applied for studying receptor binding events, creating dynamic new materials such as artificial muscles or adapted as drug delivery devices.
The tweezers described in the new study are one example of a dynamic DNA nanostructure. In earlier efforts by the Yan group, segments of DNA were successfully fashioned into tweezers, which could be activated by adding a trigger strand to the aqueous environment in which the DNA nanostructure self-assembles. This trigger strand bonds to the tweezer structure, causing the jaws of the tweezers to transition from a closed to an open position, through a property known as strand-displacement. (Figure 1 shows how strand displacement can be used to add dynamic properties to DNA structures.)
The new light-controlled tweezers, which are the first of their kind, have two key advantages. First, they open about 60 times faster than tweezers requiring an external trigger strand. Second, the new stand-alone structure could potentially be delivered into living cells and activated with light, dramatically expanding its potential applications.
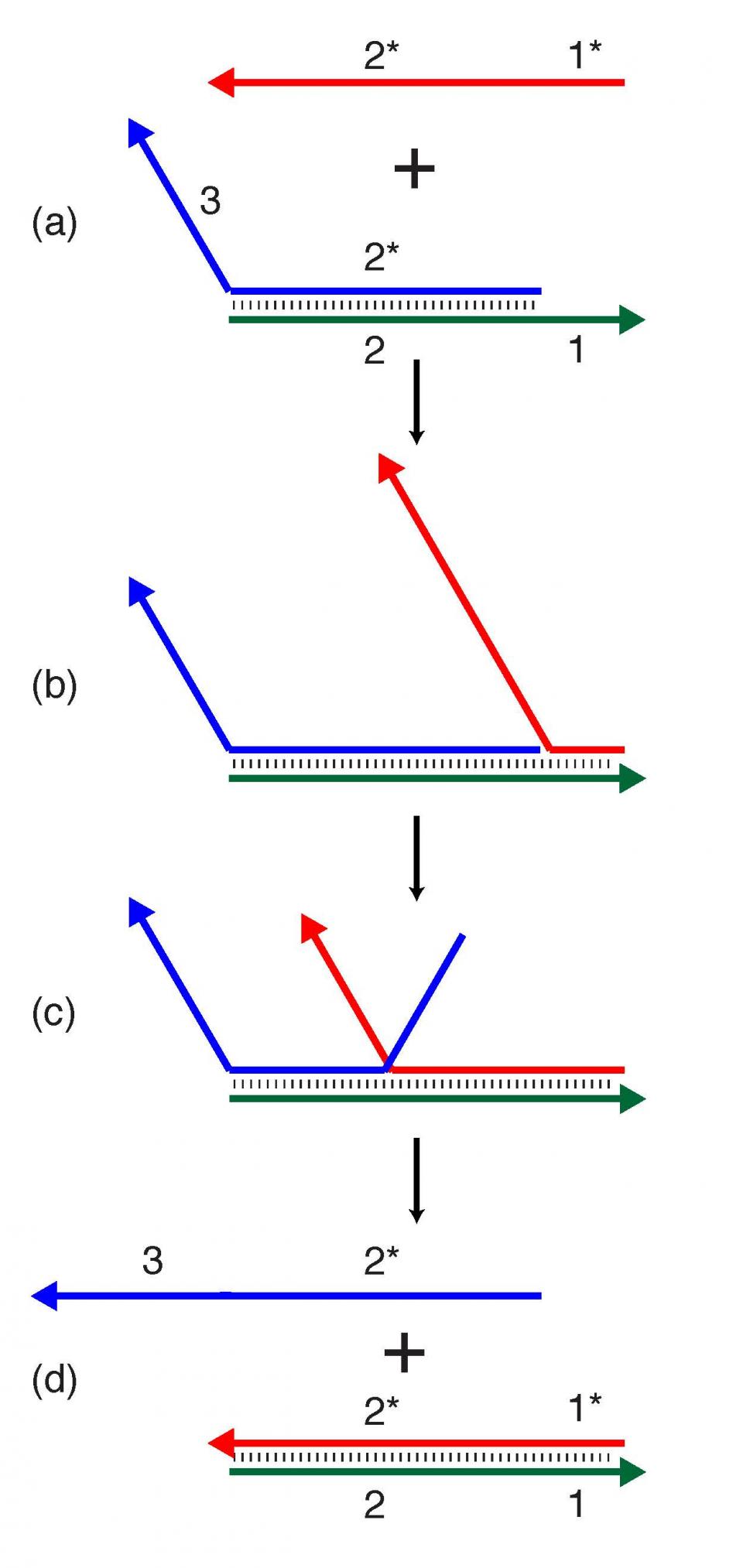
Dynamic DNA nanotechnology often makes use of toehold-mediated strand displacement reactions. In this example, the red strand binds to the single-stranded toehold region on the green strand (region 1), and then in a branch migration process across region 2, the blue strand is displaced and freed from the complex. Reactions like these are used to dynamically reconfigure or assemble nucleic acid nanostructures. The design of the new tweezers takes an innovative approach, incorporating the trigger mechanism into the nanostructure itself. In this case, the trigger strand is modified with a photocage — a chemical blocking group that prevents the trigger strand from binding with its complementary sequence. The tweezers are initially held shut by a single-stranded DNA segment. Shining ultraviolet light removes the photocage and allows the trigger strand to bind its complementary sequence, which springs open the tweezers.
More Science and technology
Extreme HGTV: Students to learn how to design habitats for living, working in space
Architecture students at Arizona State University already learn how to design spaces for many kinds of environments, and now they…
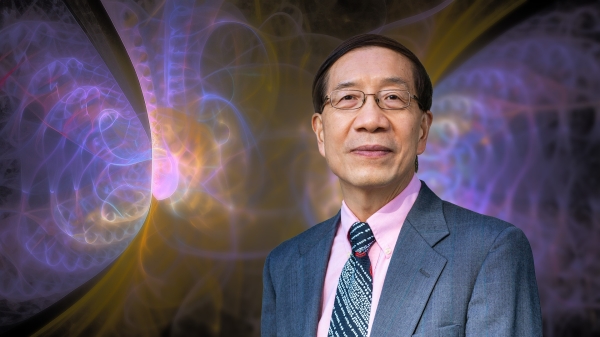
Human brains teach AI new skills
Artificial intelligence, or AI, is rapidly advancing, but it hasn’t yet outpaced human intelligence. Our brains’ capacity for…

Doctoral students cruise into roles as computer engineering innovators
Raha Moraffah is grateful for her experiences as a doctoral student in the School of Computing and Augmented Intelligence, part…