Safety first: A human-centered approach to brain implant design
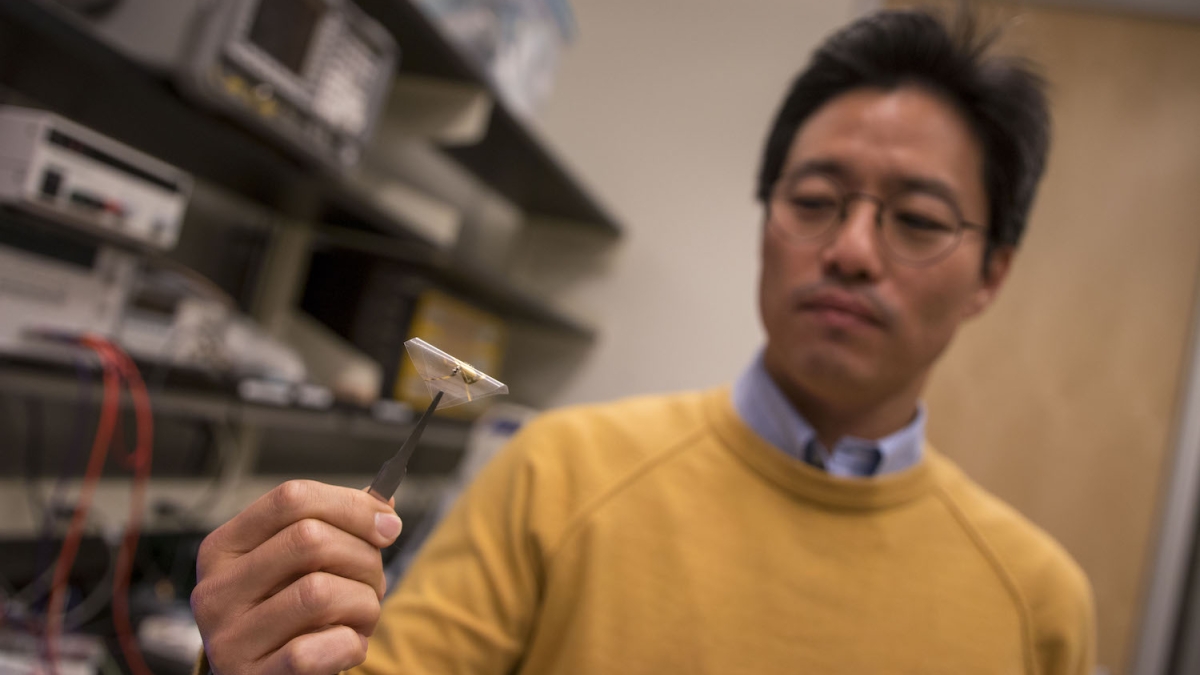
Junseok Chae. Photo by Marco-Alexis Chaira/ASU
The complex network of signals in the human brain make us capable of amazing athletic feats and innovative ideas, but they can also cause debilitating problems such as seizures. Advances in brain implant technology can help us better understand and monitor the brain’s signals and give hope to a better future for the treatment of neurological disorders.
Junseok Chae wants to use his expertise of microdevices to help people with neurological disorders through wireless, passive neural recorders — safety-minded brain implants. He believes current wired, battery-powered or energy harvesting implant technologies don’t meet the threshold of safety that patients deserve — and that he has a solution.
The Arizona State University Ira A. Fulton Schools of Engineering electrical engineering professor and associate dean for research is working to design and test a new kind of brain implant with researchers at Ohio State University and Florida International University that he hopes could one day help people who have neurological disorders such as seizures related to Parkinson’s disease.
Chae is leading the effort to build the implant prototype while his research partners test its accuracy and effectiveness in measuring brain signals.
This interdisciplinary team — whose expertise covers electrical engineering as well as signal processing and social and behavioral science — behind the project, “Fully passive and wireless multichannel neural recording for chronic in-vivo studies in animals” recently earned its latest three-year, nearly $850,000 award from the National Science Foundation.
A safer way to monitor the brain
Think of a tranquil ocean — teeming with life and activity under the surface, but seemingly calm above. What’s underwater tends to stay undetected as we look from above the surface. The brain’s activity can be similarly opaque. Brain signals are rather isolated inside our heads as the scalp, skull, and the water content, called cerebrospinal fluid, in the brain significantly attenuate, or reduce, signals from outside getting in to detect what’s going on inside.
Electroencephalogram, or EEG, technology can get through those barriers from the outside for the strongest of signals — like the sight of a whale swimming just below the ocean's surface. While good for some applications, implants are needed to detect weaker signals blocked by the brain’s outer protective layers, just as one needs to get in the water to see smaller fish.
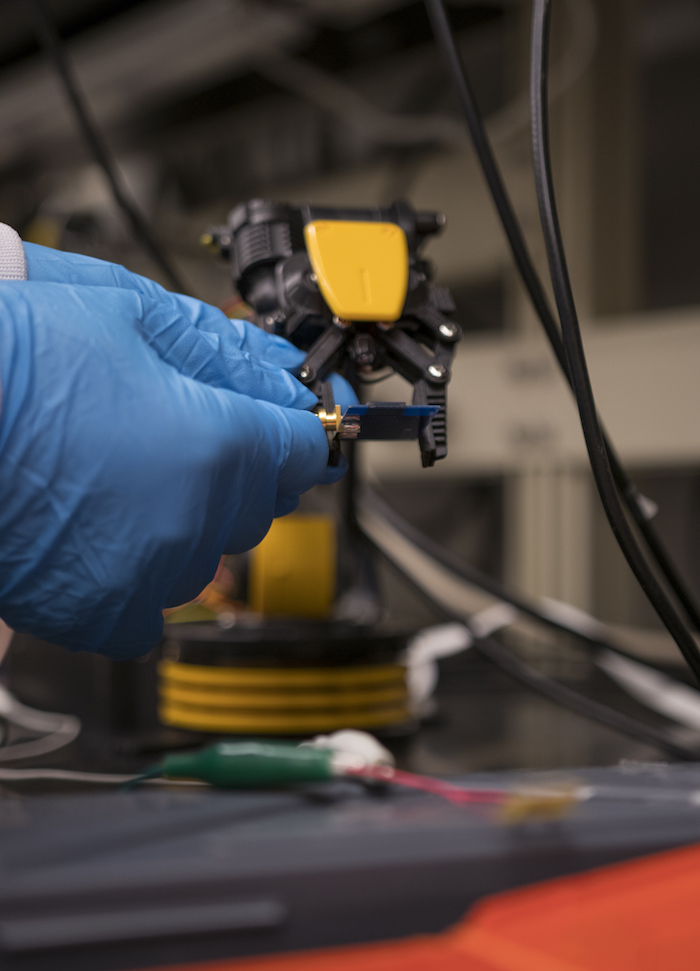
The brain implant prototype is held next to an external antenna that emits electromagnetic waves to the internal device. The electromagnetic waves reflect back with the brain signal patterns for external analysis. Photographer: Marco-Alexis Chaira/ASU
However, putting an implant in the brain currently poses many risks for a patient. Most brain implants today incorporate wires and cables to route the brain signal outside. Many research reports propose battery-powered implants to avoid wires and cables, and the battery is often located outside a patient’s head, inside the skull or under the scalp. This positioning still exposes inner tissues in a way that can lead to serious infection.
“The problem is, if a battery leaks it’s not easy to replace — who wants to go through open skull surgery just because of a battery?” Chae said.
Chae is working on a wireless design that involves fully isolated, fully passive parts so there are no points of electronics-associated failure in the implanted device.
“You don’t want to use an implant unless you have to, but when you do, wireless, fully passive [implants] shine,” Chae said. “We want to have a self-contained unit inside the skull without wires coming out or failure sources inside the brain.”
This type of device relays brain signals similar to how sonar in a fishing boat sends acoustic waves into the ocean that reflect back the presence of fish and other underwater objects.
The 4 mm x 12 mm x 0.2 mm implant design uses an external antenna that emits electromagnetic waves — the same as a mobile phone — to a tiny antenna on the implanted device. A small electronic device on the implant called a varactor then combines the incoming electromagnetic signal with brain signal and reflects both back to the external antenna in a process called backscattering.
Having a passive, wireless device in the brain helps keep complex electronics on the outside where they don’t pose a safety risk.
“I can make a complicated and humongous system outside the brain, not a problem — if that fails, it’s fine, I can fix it,” Chae said. “I just don’t want to have any complex things inside the brain.”
Backscattering is not a powered method of signal collection — it’s just a reflection of the signal that attenuates, or weakens, as it travels through the brain’s protective layers — so the device does lose some signal quality.
Chae believes the increased safety makes the compromise in performance worth it for certain applications, like seizure detection for people with Parkinson’s disease where the signals are strong enough to be detected by this wireless fully passive technology.
While such technology is likely decades away from use in humans, Chae has high hopes for what it could do to help people with neurological disorders regain their daily routines.
The external part of the system Chae speculates can be embedded in a baseball cap or clothing to conceal it, and has the potential to also deliver electrical stimulation to dampen some of the neurological disorder symptoms based on the brain signals detected.
Interdisciplinary team makes bigger impact
All these wireless and fully passive electronics are useless without the knowledge of what brain signals mean from researchers at OSU and FIU, Chae said.
Chae will lead the design of the implant’s biotelemetry, antenna and device, as well as manufacturing the implant prototype using micro electrical mechanical system, or MEMS, technology at ASU’s NanoFab facility, while his academic partners at OSU and FIU lead the testing to move the implant’s new capabilities beyond the theoretical to the experimental in animal models.
“If you don’t understand what the brain signal is for, it’s useless,” Chae said. “Without such collaboration [with OSU and FIU], the importance of the device and the telemetry and the system cannot be verified or validated.”
Building on a strong foundation
Chae has had previous success in this area with previous awards from NSF, National Institutes of Health and NASA for projects advancing innovative neurotechnologies. Five years ago, Chae earned $2 million in funding from the NSF smart-connected health program on studying single-channel wireless fully passive neural recorders. This single-channel recorder study resulted in a robust and reproducible neural recording system and protocol.
The current project, through the NSF BRAIN Initiative, is to explore a demonstration of multi-channel recording in animal models. This multi-channel recording allows effective means of monitoring brain signals, such as seizure detection, and lowers the possibilities of false positives and false negatives.
Chae’s believes his work to create a new type of medical telemetry device is why the NSF continues to show interest in his research.
“Very few wireless fully passive biotelemetry exists,” Chae said. “People have studied wireless ‘passive’ telemetry in the past, which does not have a battery, yet the active electronics on the implant require active power, which is supplied externally, such as inductive coupling.”
The reason very little wireless fully passive technology exists, Chae said, is due to a few key reasons. The dense and complex circuitry on the implant is prone to failure and requires that the external and internal implant be aligned precisely in order for it to work. Scaling the device down to a small implant is also difficult, as effective power transfer requires the implant to be relatively large.
However, Chae is hopeful the work his team has done so far and the developments they hope to achieve will help overcome these challenges as they continue their funded research.
More Science and technology

Cracking the code of online computer science clubs
Experts believe that involvement in college clubs and organizations increases student retention and helps learners build valuable…
Consortium for Science, Policy & Outcomes celebrates 25 years
For Arizona State University's Consortium for Science, Policy & Outcomes (CSPO), recognizing the past is just as important as…
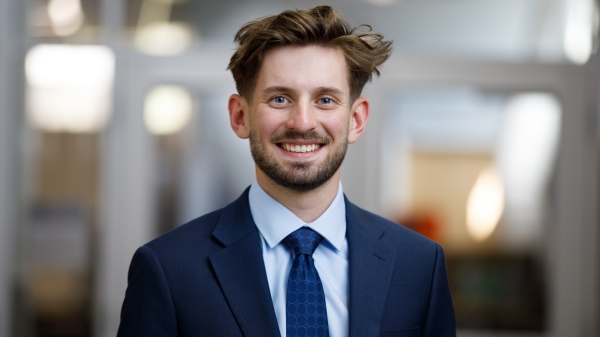
Hacking satellites to fix our oceans and shoot for the stars
By Preesha KumarFrom memory foam mattresses to the camera and GPS navigation on our phones, technology that was developed for…